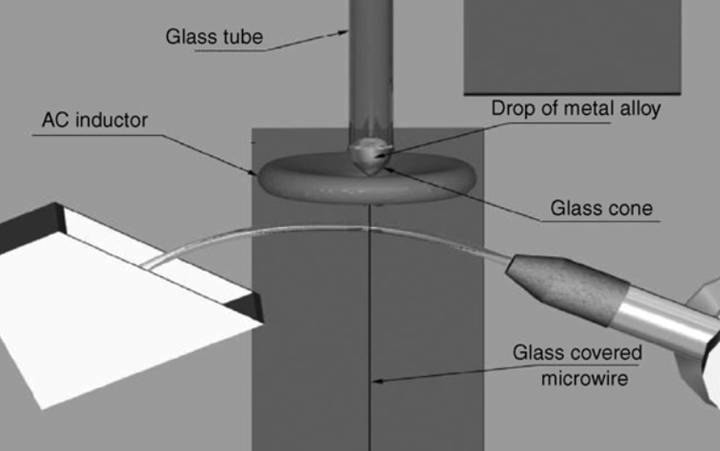
Fig.1. Schematic of the Taylor–Ulitovsky process for producing glass-coated microwires. Photo Credit: Journal of Magnetism and Magnetic Materials1
Although some types of microwire sensors and their applications in composites have been discussed previously by CW (see “Measuring temperature inside composites and bondlines” and “Contactless monitoring of temperature and pressure inside composites”), the present state-of-the-art, as well as current and future applications of magnetic microwires, are much broader.
It is worth noting that researchers in applied magnetism have worked with glass-coated microwires for decades [1-4]. Magnetic microwires are proposed for applications in many industries including: microelectronics, sensorics, security electronic surveillance, medicine, aerospace, energy harvesting/conversion, home entertainment, electrical engineering, magnetic memories, composites monitoring and more. Trends regarding these applications have been reported in several recent review papers [5-10]. One of the main applications for composites is in nondestructive monitoring of stress and temperature.
Glass-coated magnetic microwires
Soft magnetic materials, which have at least one dimension in the micrometer or nanometer size range [5-10, 14], are characterized by a high surface-area-to-volume ratio. Such materials with an amorphous, liquid-like (disordered) structure exhibit a unique combination of magnetic, mechanical (e.g., high tensile yield, better plasticity and flexibility) and anti-corrosive properties [5-10, 14], as well as being mechanically robust. This combination is promising for development of low-dimensional, cost-effective magnetic and magnetoelastic sensors and smart composites [6, 14, 17].
Amorphous magnetic materials have demonstrated versatile magnetic properties including giant magneto-impedance (GMI) effect [18] and spontaneous magnetic bistability. The principal interest in the GMI effect is related to extremely high impedance sensitivity to external stimuli, such as a magnetic field or mechanical stress. This has stimulated the development and implementation of various applications using microwires with high GMI effect, including magnetometers, smart composites and magnetoelastic sensors [18].
Spontaneous magnetic bistability is also a versatile property, and although it can be realized in properly processed amorphous ribbons [19], in various alloys of amorphous wires it is realized even without any special postprocessing [8,18]. This magnetic bistability results from a single and large Barkhausen jump between two remanent states (that is, after the external magnetic field is removed) with opposite magnetization. In other words, there is no demagnetized state in magnetically bistable materials, and they present perfectly rectangular hysteresis loops. The magnetization switching (between two remanent states) runs by fast domain wall (DW) propagation. As reported elsewhere [8], such DW propagation starts from the microwire ends, where the closure domains exist because of the demagnetizing field effect.
There are a number of methods used to produce magnetic wires that may suit particular applications [5-10,16-18], but the modified Taylor-Ulitovsky method (also known as quenching-and-drawing) (Fig. 1) allows the most extended diameter range (0.1-100 micrometers) [9]. It also enables the coating of microwires with molten glass, which improves corrosion resistance, enabling new applications in medicine, stress and temperature monitoring, construction and smart composites [5,6,17]. (Microwire manufacturing is illustrated in “Contactless monitoring of temperature and pressure inside composites”.)
Continuous casting of glass-coated microwires has been known since the 1960s [1-3] and has been shown to offer consistent microwire properties at mass production, fabrication of continuous microwire up to 10,000 meters/per bobbin, low cost and the ability to control and adjust geometrical parameters (e.g., metallic nucleus diameter and glass thickness) during fabrication [9].
Patents representing this technology include those by Tamag Iberica S.L. (Donostia-San Sebastian, Spain) and by the National Institute of Research and Development for Technical Physics (Iasi, Romania) [24-27]. Note, this method began with microwires having a non-magnetic metallic nucleus (e.g., copper, silver) and expanded to microwires with a ferromagnetic metallic nucleus in the 1970s [2,3], followed by more intensive studies in the mid-1990s [4,7].
Sensors for nondestructive monitoring
A great number of magnetic and magnetoelastic sensors have been developed from glass-coated microwires [14, 28-32], which exploit the change in magnetic properties — such as coercivity, switching field, magnetic permeability, hysteresis loop — under external stimuli including stress, temperature and magnetic field [28-32]. The main limitation of such sensor technology is relatively low signal levels, and hence the distance from the microwire to the detecting system is usually limited to several millimeters. Use of thicker wires or smart electronics (e.g, preamplifiers, registration facilities) can increase this detection distance to several centimeters [32].
A different concept has been developed based on embedding into advanced composites magnetic wires with tunable magnetic permittivity at the gigahertz range to avoid interrogation distance limitations [14,33-36]. This is attractive, as the demand for real-time monitoring of local stresses and temperature in composites is growing. Recently developed composites containing piezoelectric fibers with diameters of 10-100 micrometers present significant advantages over conventional piezoelectric actuators and have thus become promising technology for nondestructive monitoring [37]. However, their operation requires electrical field supply plates, which occupy a significant area.
Alternatively, the use of ferromagnetic microwires with high-frequency magneto-impedance quite sensitive to applied stress, temperature and magnetic field embedded into composites [33-36, 38-40], allows remote stress, temperature and magnetic field monitoring using free-space microwave spectroscopy. This is possible because the effective permittivity of magnetic wire arrays shows strongly tunable behavior [34-36]. In fact, it has been demonstrated experimentally that composites with embedded ferromagnetic microwires exhibit a frequency region at the microwave regime with scattering spectra strongly dependent on external stimuli — including applied magnetic field and applied stress [38-40].
Fig. 2. Principle of tunable composites with embedded magnetic microwires. Photo Credit: Dr. Arkady Zhukov “Giant Magnetoimpedance Effect of Magnetically Soft Microwires for Sensor Applications”
Fig. 3. Schematic of a free-space measuring setup allowing remote characterization of composites with embedded microwires. Photo Credit: Dr. Arkady Zhukov “Giant Magnetoimpedance Effect of Magnetically Soft Microwires for Sensor Applications”
The dependence of the effective permittivity and transmission parameters of such smart composites on external stimuli in the gigahertz range indicate that composites with embedded microwires can be useful for structural health monitoring (SHM) and self-sensing applications [38-40] and can be tunable (Fig. 2). An experimental setup to measure reflection (R) and transmission (T) parameters of such tunable composites is shown in Fig. 3.
The implementation of such a technology requires a microwire with stress-sensitive magnetic properties. As-prepared and stress-annealed microwires have indeed shown change in impedance with torsion or tensile stress [41,42]. Further, stress dependence of the transmission coefficient has been measured in the free-space near field of a composite with embedded microwires [38].
Demonstration in remote monitoring of polymerization process
Recently, this technique was employed to monitor a resin polymerization process. The free-space remote monitoring system shown in Fig. 3 was used to obtain the in-situ measurements shown in Figs. 4, 5 and 6. In these experiments, T and R parameters were measured from cobalt-rich microwires (i.e., Fe3.8Co65.4Ni1B13.8Si13Mo1.35C1.65) embedded in a polymerizing matrix [43].
Fig. 4. Evolution of temperature during resin polymerization measured using a thermocouple. Photo Credit: Composites Part A: Applied Science and Manufacturing3
By independent direct measurements using a standard thermocouple, it was observed that the polymerization produces heating of the matrix up to 80°C after ≈20 minutes, followed by cooling down to almost ambient temperature at ≈50 minutes, when T≈30°C (Fig. 4).
Microwires can also capture the mechanical stresses that accompany the change in matrix density during polymerization and shrinkage as it cools. Generally, these internal stresses are complex and non-homogeneously distributed inside the matrix [44]. Fig. 5a shows considerable variation of T observed in the range of 4-7 gigahertz. Change in the microwire magnetic properties is also reflected by the change in the measured hysteresis loop during polymerization (Fig. 5b). The compressive origin of these stresses is confirmed by the measured hysteresis loop of the microwires under applied stress as shown in Fig. 6, noting the hysteresis maintains a linear shape under applied tensile stress. The magnetic anisotropy field, Hk, of the microwires increases with application of tensile stress and this is the opposite to that observed in Fig. 5b
Fig. 5. Evolution of the transmission (T) parameter of a polymer with microwire inclusions measured using a free-space monitoring system (a) and hysteresis loops of a Fe3.8Co65.4Ni1B13.8Si13Mo1.35C1.65 microwire array (b) during resin polymerization. Photo Credit: Composites Part A: Applied Science and Manufacturing3
Fig. 6. Evolution of hysteresis loops of Fe3.8Co65.4Ni1B13.8Si13Mo1.35C1.65 microwire under applied tensile stress. Photo Credit: Composites Part A: Applied Science and Manufacturing3
These experimental results confirm the feasibility of magnetic microwires for in-situ monitoring of matrix polymerization that involves change of temperature and stress in composites. The same technique can be used for remote monitoring not only during the polymerization, but also for SHM during in-service use.
Continued sensor development
Currently, the University of The Basque Country (San Sebastian, Spain) and Tamag Iberica Ltd. are involved in the EU-funded Horizon project “Aerospace Composites Digitally Sensorized from Manufacturing to End-Of-Life (INFINITE).” This consortium has also been involved in several European, national and regional projects on the same topic, including:
- “Development of soft magnetic microwires … for micro-sensors” (SoMaMicSens, manunet.net)
- RTM4.0, “Composites for automobiles fabricated by RTM adapted to a philosophy of Industry 4.0”
- “Innovative sensors based on multifunctional active materials for 4.0 monitoring of construction processes and structural health in infrastructures”
- “New functional micro- and nano-materials structured for spintronics and devices, actuators, sensors and magnetic memories”
- “Development of magnetic microwires for microsensors of interest to the railway sector”
Glass-coated, amorphous microwires combine nanometer to micrometer diameters — enabling embedding into composites without degrading mechanical properties — combined with excellent soft magnetic properties, biocompatibility for medical implants, high mechanical properties and improved corrosion resistance, making them well suited as sensors for high-performance and high-value applications, as well as for everyday products. For a full list of references, contact arkadi.joukov@ehu.eus.
Image References
1 V.S Larin, A.V Torcunov, A Zhukov, J González, M Vazquez, L Panina,
“Preparation and properties of glass-coated microwires,” Journal of Magnetism and Magnetic Materials, Vol. 249, 2002, pp 39-45.
2V. Zhukova, P. Corte-Leon, L. González-Legarreta, A. Talaat, J. M. Blanco, M. Ipatov, J. Olivera and A. Zhukov, “Review of Domain Wall Dynamics Engineering in Magnetic Microwires,” Nanomaterials 10, 2020) 2407, pp. 7-22
3 A. Allue, P. Corte-León, K. Gondra, V. Zhukova, M. Ipatov, J. M. Blanco, J. Gonzalez, M. Churyukanova, S. Taskaev, A. Zhukov, “Smart composites with embedded magnetic microwire inclusions allowing non-contact stresses and temperature monitoring,” Composites Part A: Applied Science and Manufacturing, Vol. 120, 2019, pp 12-20.
About the Author
Arkady Zhukov
Dr. Arkady Zhukov earned a Ph.D. from the Institute of Solid State Physics (Chernogolovka) at the Russian Academy of Science in 1988 and a Doctor of Science (habilitation) from Moscow State “Lomonosov” University in 2010. Since 2011, he’s served as an Ikerbasque (Basque Foundation for Science) research professor in the advanced polymers and materials department at the University of the Basque Country, Spain. He has published more than 600 papers in international journals, given more than 100 plenary, keynote or invited talks at international conferences and is an associate editor of IEEE Magnetic letters and International Journal on Smart Sensing and Intelligent Systems. In 2000, he founded the spin-off company, Tamag Iberica S.L., in which he serves as scientific supervisor.
Related Content
Multi-scale 3D CT imaging enables digital twinning, high-fidelity simulation of composite structures
Computed tomography (CT) provides highly accurate 3D analysis of internal microstructure, performance simulation of carbon fiber/PEEK satellite strut.
Read MoreComposite prepreg tack testing
A recently standardized prepreg tack test method has been developed for use in material selection, quality control and adjusting cure process parameters for automated layup processes.
Read MoreNotched testing of sandwich composites: The sandwich open-hole compression test
A new ASTM-standardized open-hole compression test method seeks to determine the notch sensitivity of sandwich composites.
Read MoreDamage tolerance testing of sandwich composites: The sandwich flexure-after-impact (FAI) test
A second new ASTM-standardized test method assesses the damage tolerance of sandwich composites under flexural loading.
Read MoreRead Next
Sensors: Data for next-gen composites manufacturing
In the quest for sustainability, sensors are reducing cycle times, energy use and waste, automating closed-loop process control and increasing knowledge to open new possibilities for intelligent manufacturing and structures.
Read MoreCW’s 2024 Top Shops survey offers new approach to benchmarking
Respondents that complete the survey by April 30, 2024, have the chance to be recognized as an honoree.
Read MoreComposites end markets: Energy (2024)
Composites are used widely in oil/gas, wind and other renewable energy applications. Despite market challenges, growth potential and innovation for composites continue.
Read More