Visibility into processes
Sensors at left (top to bottom): Heat flux (TFX), in-mold dielectric (Lambient), ultrasonic (Univ. of Augsburg), disposable dielectric (Synthesites) and microwire (AvPro) in between penny and thermocouple. Graphs (top, clockwise): Collo perittivity (CP) versus Collo ion viscosity (CIV) using electromagnetic sensor, resin resistance versus time (Synthesites) and digital model of caprolactam injection into preform (CosiMo project, DLR ZLP, University of Augsburg).
As global industry continues to emerge from the COVID-19 pandemic, it has pivoted to prioritize sustainability, which demands reduction of waste and resource consumption (e.g., energy, water and materials). Thus, manufacturing must become more efficient and intelligent. But this requires information. For composites, where does this data come from?
Defining the measurements required to improve part quality and production, and the sensors required to achieve those measurements, is one of the first steps toward intelligent manufacturing, as described in CW’s 2020 series of articles on Composites 4.0. Throughout 2020 and 2021, CW has reported on sensors — dielectric sensors, thermal flux sensors, fiber optic sensors and contactless sensors that use ultrasound and electromagnetic waves — as well as projects that demonstrate their capabilities (see CW’s Sensors content collection online). This article draws from this coverage to discuss the sensors being used in composites, their promised benefits and challenges and the landscape of technology being developed. Notably, companies that are becoming the leaders of the composites industry have already begun to explore and navigate this landscape.
Why use sensors?
Network of sensors in CosiMo
A network of 74 sensors — 57 were ultrasonic sensors developed by the University of Augsburg (shown at right as light blue dots in upper and low mold halves) — were used in the T-RTM molding of a thermoplastic composite battery box cover demonstrator for the CosiMo project. Photo Credit: CosiMo project, DLR ZLP Augsburg, University of Augsburg
Goal #1: Save money. CW’s December 2021 blog, “Customizing ultrasonic sensors for composites process optimization and control,” described the University of Augsburg’s (UNA, Augsburg, Germany) work developing a network of 74 sensors used to manufacture an electric vehicle battery box cover demonstrator for the CosiMo (composites in smart mobility) project. The part was made using thermoplastic resin transfer molding (T-RTM), which in-situ polymerizes a caprolactam monomer into a polyamide 6 (PA6) composite. Markus Sause, a UNA professor and director of the Augsburg Artificial Intelligence (AI) Production Network at UNA, explains why sensors are important: “The biggest advantage we are offering is to visualize what’s going on inside the black box during processing. Currently, most manufacturers only have limited systems to make that happen. For example, they are using very simple or specific sensors when fabricating large aerospace parts using resin infusion. And if the infusion process goes wrong, you basically have a large piece of scrap. But if you have a solution to understand what goes wrong during production and why, you can then address and correct it, and save a lot of money.”
Thermocouples are one example of a “simple or specific sensor,” used for decades to monitor temperature in composite laminates during autoclave or oven cure. They have even been used to control the temperature in an oven or in a heat blanket to cure a composite repair patch using a hot bonder. Resin manufacturers have used various sensors in the lab to monitor change in resin viscosity with time and temperature to develop cure recipes. What is emerging, however, is a network of sensors that can visualize and control the manufacturing process in-situ, based on multiple parameters (e.g., temperature and pressure) and the state of the material (e.g., viscosity, polymerization, crystallization).
For example, the ultrasonic sensor developed for the CosiMo project uses the same principle as ultrasonic testing that has become the mainstay for non-destructive inspection (NDI) of finished composite parts. “Our aim was to minimize the time and labor required for post-production inspection on future components as we move toward digital manufacturing,” says Petros Karapapas, principal engineer at Meggitt (Loughborough, U.K.). He worked with the National Composites Centre (NCC, Bristol, U.K.) to demonstrate use of linear dielectric sensors developed by Cranfield University (Cranfield, U.K.) to monitor flow and cure of Solvay (Alpharetta, Ga., U.S.) EP 2400 epoxy during RTM of a 1.3 meter-long, 0.8-meter-wide and 0.4-meter-deep composite housing for a commercial aeroengine heat exchanger. “As we look at how to make larger components and at higher production rates, we cannot afford to do all of the conventional post-processing inspection and testing for each part,” says Karapapas. “Right now, we make test panels alongside such RTM parts and then we do mechanical tests to validate the cure cycle. But with this sensor, that won’t be necessary.”
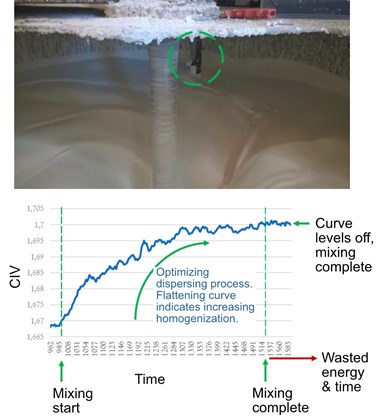
Collo Probe submerged (green circle at top) in paint mixing vessel detects when mixing is complete, saving time and energy. Photo Credit: ColloidTek Oy
“We are not aiming to be another lab device, but instead are focused on systems for production,” says Matti Järveläinen, CEO and founder of ColloidTek Oy (Collo, Tampere, Finland). CW’s January 2022 blog, “Fingerprinting liquids for composites,” explored Collo’s combination of electromagnetic field (EMF) sensors, signal processing and data analytics to measure the “fingerprint” of any liquid, such as a monomer, resin or adhesive. “What we are offering is a new technology to provide direct feedback in real time, so that you can better understand how the process actually works and can react if something goes wrong,” says Järveläinen. “Our sensors convert real-time data into physical quantities that are understandable and actionable, like rheological viscosity, which allows optimizing the processes. For example, you can shorten mixing time because you can clearly see when mixing is complete. So, you can improve productivity, save energy and reduce scrap versus processing that is less optimized.”
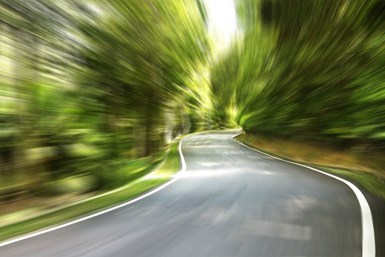
Photo Credit: Getty Images
Goal #2: Increase process knowledge and visualization. In the case of processes such as polymerization, says Järveläinen, “you cannot see much from a snapshot only. You are just taking a sample and going into the lab and seeing what the state was minutes or hours ago. It’s like driving on a motorway and just opening your eyes for one minute each hour and trying to predict where the road goes from that.” Sause agrees, noting the sensor network developed in CosiMo “helped us to have full visibility into the process and material behavior. We could see the local effects in the process in response to variations in part thickness or in the integrated materials, like the foam core. What we’re trying to do is provide information about what is actually occurring in the mold. And this allows us to determine various things such as the shape of the flow front, the time for it to reach each part of the preform and the degree of polymerization at each sensor location.”
Collo has worked with manufacturers of epoxy adhesive, paint and even beer to create a process profile for each batch produced. Each manufacturer can now see the dynamics of its process and set more optimal parameters, with alarms to enable intervention when the batch is out of spec. This helps to stabilize and improve quality.
Video of the flow front in the CosiMo part (injection inlet is white dot at center) as a function of time based on the measurement data from the network of in-mold sensors. Photo Credit: CosiMo project, DLR ZLP Augsburg, University of Augsburg
“I want to know what’s going on inside the part manufacturing process while it’s happening, as opposed to opening the box and seeing what happened afterwards,” says Karapapas at Meggitt. “What we developed using the dielectric sensor from Cranfield gave us eyes on the process in-situ, and we were also able to validate cure of the resin.” Monitoring of cure/polymerization and resin flow is possible with all six types of sensors described below (not an exhaustive list, but a just a small selection, as are the suppliers). Some sensors have additional capabilities, and combining sensor types can expand what is possible to track and visualize during composites molding. This was demonstrated during CosiMo, which used ultrasonic, dielectric and piezoresistive in-mold sensors provided by Kistler (Winterthur, Switzerland) for temperature and pressure measurement.
Goal #3: Reduce cycle time. Collo sensors can measure the homogeneity of two-part, snap-cure epoxy as parts A and B are mixed and injected during RTM and at every location in the mold where such sensors are placed. This could help to qualify faster cure resins for applications such as urban air mobility (UAM), which would offer much faster cure cycles versus current one-component epoxies like RTM6.
Collo sensors can also monitor and visualize an epoxy resin being degassed, infused and cured, as well as when each process is complete. Ending cure and other processes based on the actual state of the material being processed — versus a legacy time and temperature recipe — is called material state management (MSM). Pursued for decades by companies like AvPro (Norman, Okla., U.S.), MSM tracks variations in the part’s materials and process as it pursues specific targets for glass transition temperature (Tg), viscosity, polymerization and/or crystallization. The sensor network and digital analysis in CosiMo, for example, was used to determine the minimum amount of time required in the heated RTM press and mold, finding that a maximum polymerization of 96% was achieved in 4.5 minutes.
Suppliers of dielectric sensors such as Lambient Technologies (Cambridge, Mass., U.S.), Netzsch (Selb, Germany) and Synthesites (Uccle, Belgium) have also demonstrated their ability to shorten cycle times. Synthesites reported from R&D projects with composites manufacturers Hutchinson (Paris, France) and Bombardier Belfast, now Spirit AeroSystems (Belfast, Ireland), that it was able to reduce the cure cycle for RTM6 by 30-50% based on real-time measurements of resin electrical resistance and temperature, which are converted into estimated viscosity and Tg by its Optimold data acquisition unit and Optiview software. “The manufacturer can see the Tg in real time, so they can decide when to stop the cure cycle,” explains Synthesites director Nikos Pantelelis. “They don’t have to wait to complete a longer-than-necessary legacy cycle. For example, the legacy cycle for RTM6 is 2 hours at 180°C for full cure. We’ve seen that in certain geometries this can go down to 70 minutes.” This has also been demonstrated in the INNOTOOL 4.0 project (see “Speeding RTM with heat flux sensors”), where thermal flux sensors were used to reduce the RTM6 cure cycle from 120 to 90 minutes.
Goal #4: Closed-loop control for adaptive processes. For the CosiMo project, the ultimate quest was to enable automated, closed-loop control during composites part production. This was also the goal for the ZAero and iComposite 4.0 projects (as was a 30-50% cost reduction) which CW reported on in 2020. Note that these dealt with different processes — automated placement of prepreg tape (ZAero) and fiber spray preforming for RTM with snap-cure epoxy (iComposite 4.0) compared to the high-pressure T-RTM in CosiMo. All of these projects used sensors with digital models and algorithms to simulate the process and predict outcomes for the finished part.
Sause explains that process control can be envisioned as a series of steps. The first step is to have the sensors and process equipment integrated, he says, “to visualize what's happening inside your black box of processing and what parameters to use. Another few steps, perhaps halfway to closed-loop control, is to have the ability to hit the stop button in order to intervene, adapt the process and prevent reject parts. As a final step,you can develop the digital twin, which enables automation but also requires investment in a machine learning approach.” In CosiMo, this investment allowed the sensors to feed data to the digital twin, where edge analytics (calculations performed at the edge of the process line versus from a central data repository) were then used to predict flow front dynamics, the fiber volume content of each textile preform and potential dry spots. “Ideally, you can establish settings to enable closed-loop control and adjustment during the process,” says Sause. “These would include parameters like injection pressure, mold pressure and temperature. You can also use this information to optimize your materials.”
On the way to that goal, companies are using sensors to automate processes. For example, Synthesites is working with its customers to integrate sensors with equipment so that resin inlets are closed when infusion is completed or a heated press is opened when target cure has been reached.
Selecting and integrating sensors
To determine which sensors are best for each use case, notes Järveläinen, “you need to understand what variations in material and process you want to monitor, and then you must have an analyzer.” An analyzer takes the raw data collected by the interrogator or data acquisition unit and converts it into usable information for the manufacturer. “You actually see many companies that have sensors integrated, but then they don’t do anything with the data,” says Sause. What is needed, he explains, is “systematic data acquisition, and also a data storage architecture, to be able to do something with the data.”
“End-users don’t just want to look at raw data,” says Järveläinen. “They want to know, ‘is the process optimized?’ and ‘when can it proceed to the next step?’ For that, you need to do analytics incorporating multiple sensors and then use machine learning, which speeds up the process.” This edge analytics and machine learning approach, used by Collo and the team in CosiMo, enables visualization via graphs of viscosity, digital models of the resin flow front and, ultimately, the ability to control process parameters and machinery.
Optimold is an analyzer that Synthesites has developed for its dielectric sensors. Controlled by Synthesites’ Optiview software, an Optimold unit uses temperature and resin resistance measurements to make calculations and show real-time graphs monitoring the resin state, including mix ratio, chemical aging, viscosity, Tg and degree of cure. It can be used with prepreg and liquid molding processes. A separate unit, Optiflow, is used for flow monitoring. Synthesites has also developed a Cure Simulator, which requires no cure sensors in the mold or part, but instead uses temperature sensors and a resin/prepreg sample in this analyzer unit. “We are applying this latest approach for infusion and adhesive curing for wind turbine blade production,” says Synthesites director Nikos Pantelelis.
Synthesites process control systems integrate sensors, Optiflow and/or Optimold data acquisition units and OptiView and/or Online Resin State (ORS) software. Photo Credit: Synthesites, edited by CW
Thus, most sensor suppliers have developed their own analyzers, and some use machine learning while others do not. But it is also possible for composites manufacturers to develop their own customized systems or buy off-the-shelf instruments and modify them to meet specific needs. However, analyzer capability is just one factor to consider. There are many others.
Contact is also an important consideration when selecting which sensors to use. Sensors may require contact with the material, the interrogator or both. For example, heat flux and ultrasonic sensors can be inserted into an RTM mold at 1-20 millimeters from the surface — contact with the material in the mold is not necessary for accurate monitoring. Ultrasonic sensors can also interrogate into the part at different depths based on the frequency used. Collo electromagnetic sensors can read into the depths of a liquid or part as well — 2-10 centimeters, depending on the interrogation frequency — and through a non-metallic container or tool in contact with the resin.
However, magnetic microwire (see “Contactless monitoring of temperature and pressure inside composites”) is currently the only sensor able to interrogate up to 10 centimeters away from the composite. This is because it uses electromagnetic induction to elicit a response from the sensor, which is embedded in the composite. AvPro’s ThermoPulse microwire sensor, embedded in an adhesive bondline, has been interrogated through a 25-millimeter-thick carbon fiber laminate to measure temperature during bonding. Due to microwire’s hair-like diameter of 3-70 micrometers, it does not affect composite or bondline properties. At a slightly larger diameter of 100-200 micrometers, fiber optic sensors can also be embedded without reducing structural properties. However, because they measure using light, fiber optic sensors must have a wired connection to the interrogator. Similarly, because dielectric sensors use electric voltage to measure a resin’s properties, they too must be wired to the interrogator, and most also must contact the resin they are monitoring.
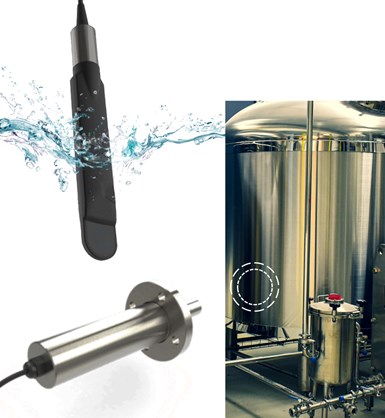
Collo Probe (top) sensors can be immersed into a liquid while a Collo Plate (bottom) is installed into the wall of containment/mixing vessels or process pipes/feed lines. Photo Credit: ColloidTek Oy
Temperature capability of sensors is another key consideration. For example, most off-the-shelf ultrasonic sensors typically operate at temperatures up to 150°C, but the part in CosiMo required molding at more than 200°C. Thus, UNA had to design an ultrasonic sensor with that capability. Lambient’s disposable dielectric sensors can be used on a part’s surface up to 350°C and their reusable, in-mold sensors can be used up to 250°C. RVmagnetics (Košice, Slovakia) has developed its microwire sensor for composites to withstand a 500°C cure. Although the Collo sensor technology itself has no theoretical temperature limitation, says Järveläinen, the tempered glass shielding for Collo Plate and a new polyetheretherketone (PEEK) housing for Collo Probe are both being tested for continuous service at 150°C. Meanwhile, PhotonFirst (Alkmaar, Netherlands) used a polyimide coating to provide a 350°C service temperature for its fiber optic sensors used in the SuCoHS project for sustainable and cost-effective high-temperature composites.
Another factor to consider, especially for installation, is whether a sensor measures at a single point or is a linear sensor with multiple sensing points. For example, Com&Sens (Eke, Belgium) fiber optic sensors can be up to 100 meters long with as many as 40 fiber bragg grating (FBG) sensing points at a minimum 1-centimeter spacing. These sensors have been used for structural health monitoring (SHM) in 66-meter-long composite bridges as well as for monitoring resin flow during infusion of large bridge decks. Installing separate point sensors for such projects would require myriad sensors plus significant installation time. The NCC and Cranfield University claim a similar benefit for their linear dielectric sensor. Compared to the single-point dielectric sensors supplied by Lambient, Netzsch and Synthesites, says Jack Alcock, Technology Pull-Through (TPT) program manager at the NCC, “for our linear sensors, we can monitor resin flow continuously along a length, which significantly reduces the number of sensors you need in your part or tool.”
AFP of fiber optic sensors
NLR integrated a special unit into lane eight of a Coriolis AFP head to place four fiber optic sensor arrays into a high-temperature, carbon fiber-reinforced composite test panel. Photo Credit: SuCoHS project, NLR
Linear sensors are also facilitating automated installation. In the SuCoHS project, Royal NLR (Netherlands Aerospace Centre, Marknesse) developed a special unit, integrated into lane eight of a Coriolis Composites (Queven, France) automated fiber placement (AFP) head to embed four arrays (separate fiber optic lines), each with five to six FBG sensors (23 sensors total supplied by PhotonFirst), in a carbon fiber test panel. RVmagnetics has placed its microwire sensor into pultruded GFRP rebar. “The wires are discontinuous [most microwires for composites are 1-4 centimeters long] but are placed automatically on a continuous basis as the rebar is produced,” says RVmagnetics cofounder Ratislav Varga. “You have a coil with 1 kilometer of microwire and feed it into the rebar production equipment, without changing how the rebar is made.” Meanwhile, Com&Sens is working on automated techniques for embedding fiber optic sensors during filament winding of pressure vessels.
Carbon fiber can cause an issue with dielectric sensors due to its ability to conduct electricity. Dielectric sensors use two electrodes placed in close proximity to each other. “If the fibers bridge the electrodes they will short-circuit the sensors,” explains Lambient founder Huan Lee. In this case, a filter is used. “The filter lets resin through the sensors but insulates them from the carbon fiber.” The linear dielectric sensor developed by Cranfield University and the NCC uses a different approach, comprising two twisted copper wires. When voltage is applied, an electromagnetic field is created between the wires, which is used to measure resin impedance. The wires are coated with an insulating polymer which does not affect generating the electric field, yet prevents short circuits from carbon fiber.
Cost, of course, is also a concern. Com&Sens cites an average cost of €50-125 per FBG sensing point, which could come down to roughly €25–35 if applied in volume — e.g., to 100,000 pressure vessels. (This is a fraction of the current and projected production capacity for composite pressure vessels, see CW’s 2021 articles on hydrogen.) Karapapas at Meggitt says quotes he received for fiber optic lines with FBG sensors averaged £250/sensor (≈€300/sensor) and interrogators were roughly £10,000 (€12,000). “The linear dielectric sensor we’ve tested is more a coated wire you can buy off the shelf,” he adds. “The interrogator that we use,” adds Alex Skordos, reader (senior researcher) in Composites Process Science at Cranfield University, “is an impedance analyzer, which is very accurate and costs at least £30,000 [≈€36,000], but the NCC uses a far simpler interrogator that comprises essentially off-the-shelf modules from the commercial company Advise Deta [Bedford, U.K.].” Synthesites quotes €1,190 for in-mold sensors, €20 for disposable/on-part sensors, €3,900 for Optiflow and €7,200 for Optimold, with an escalating discount for multiple analyzer units. These prices include the Optiview software and any support necessary, says Pantelelis, adding that wind blade manufacturers are saving 1.5 hours per cycle, enabling an additional blade per month per line and 20% reduced energy usage for an ROI of just four months.
New possibilities, path forward
Companies that use sensors will gain an advantage as Composites 4.0 digital manufacturing moves forward. For example, says Grégoire Beauduin, Com&Sens business development director, “as pressure vessel manufacturers try to decrease weight, material usage and cost, they can use our sensors to prove their designs and for monitoring production as they ramp to meet required volumes by 2030. The same sensors used to assess strain levels within the plies during filament winding and cure can also monitor the tank’s integrity during thousands of refueling cycles, predict needed maintenance and enable recertification at the end of design life. We can provide a digital twin data-pool for every composite pressure vessel produced, and this solution is also being developed for satellites.”
Enabling digital twin and thread
Com&Sens is working with composite manufacturers to use its fiber optic sensors to enable a flow of digital data through design, production and service (right) to support a digital ID card (left) that supports the digital twin for each part manufactured. Photo Credit: Com&Sens and Fig. 1 in “Engineering Design with Digital Thread” by V. Singh, K. Wilcox.
Thus, sensor data enables digital twins, but also the digital thread spanning design, production, in-service operation and end-of-life. When analyzed using AI and machine learning, these data feed back into design and processing, enabling improved performance and sustainability. This also changes how supply chains can cooperate. For example, adhesive producer Kiilto (Tampere, Finland) uses Collo sensors to help its customers control the proportions of components A, B, etc., in their multi-component adhesive mixing equipment. “Kiilto can now tune the composition of its adhesives for individual customers,” says Järveläinen, “but it also allows Kiilto to see how the resin is interacting in its customer’s process and how its customer is interacting with its products, and this is changing how the supply chain can work together.”
OPTO-Light used Kistler, Netzsch and Synthesites sensors, monitoring cure to achieve thermoplastic overmolded epoxy CFRP parts. Photo Credit: AZL
Sensors are also enabling innovative new material and process combinations. Described in CW’s 2019 article on the OPTO-Light project (see “Thermoplastic overmolded thermosets, 2-minute cycle, one cell”), AZL Aachen (Aachen, Germany) used a two-step process to horizontally compression mold unidirectional (UD) carbon fiber/epoxy prepreg and then overmold with 30% short glass fiber-reinforced PA6. The key was to only partially cure the prepreg so that remaining reactivity in the epoxy could achieve attachment with the thermoplastic. AZL used Optimold and Netzsch DEA288 Epsilon analyzers with Synthesites and Netzsch dielectric sensors as well as Kistler in-mold sensors and DataFlow software to optimize injection molding. “You must look inside the prepreg compression molding process because knowledge about the state of cure must be sure in order to achieve a good join with the thermoplastic overmolding,” explains AZL research engineer Richard Schares. “In the future, the process may be adaptive and intelligent, with process pivoting triggered by the sensor signals.”
However, there is a fundamental problem, says Järveläinen, “which is the customer’s lack of knowledge regarding how to integrate these various sensors into their processes. Most don't have sensor experts in their companies.” For now, the path forward requires a back-and-forth exchange of information between the sensor manufacturer and the customer. And there are organizations like AZL, DLR (Augsburg, Germany) and the NCC that are developing multi-sensor expertise. Sause says there are groups within UNA and also spin-off companies that can provide sensor integration and digital twin services. The Augsburg AI Production Network has rented a 7,000-square-meter facility for that very purpose, he adds, “extending the development blueprint from CosiMo to a very broad scale, including automated cells linked together where partners from industry can place machines, run projects and see how to integrate new AI solutions.”
Meggitt’s dielectric sensor demonstration with the NCC was just such a first step, says Karapapas. “Eventually, I want to monitor my processes and workflow and feed that into our ERP system so that I can know in advance what components to make, what people I need and what materials to order. We have now started that evolution toward digitized automation.”
Related Content
Recycling end-of-life composite parts: New methods, markets
From infrastructure solutions to consumer products, Polish recycler Anmet and Netherlands-based researchers are developing new methods for repurposing wind turbine blades and other composite parts.
Read MoreInfinite Composites: Type V tanks for space, hydrogen, automotive and more
After a decade of proving its linerless, weight-saving composite tanks with NASA and more than 30 aerospace companies, this CryoSphere pioneer is scaling for growth in commercial space and sustainable transportation on Earth.
Read MoreHexagon Purus opens new U.S. facility to manufacture composite hydrogen tanks
CW attends the opening of Westminster, Maryland, site and shares the company’s history, vision and leading role in H2 storage systems.
Read MoreMingYang reveals 18-MW offshore wind turbine model with 140-meter-long blades
The Chinese wind turbine manufacturer surpasses its 16-MW platform, optimizes wind farm construction costs for 1-GW wind farms.
Read MoreRead Next
Discover More About Sensors for Composites Processing
Throughout 2020 and 2021, CW has reported on sensors that can be used to process composites, as well as projects that demonstrate their installation and capabilities. This microsite collates CW’s reporting on sensors to provide, in one place, information about the types of sensors being used in composites.
Read MoreDeveloping bonded composite repair for ships, offshore units
Bureau Veritas and industry partners issue guidelines and pave the way for certification via StrengthBond Offshore project.
Read More“Structured air” TPS safeguards composite structures
Powered by an 85% air/15% pure polyimide aerogel, Blueshift’s novel material system protects structures during transient thermal events from -200°C to beyond 2400°C for rockets, battery boxes and more.
Read More