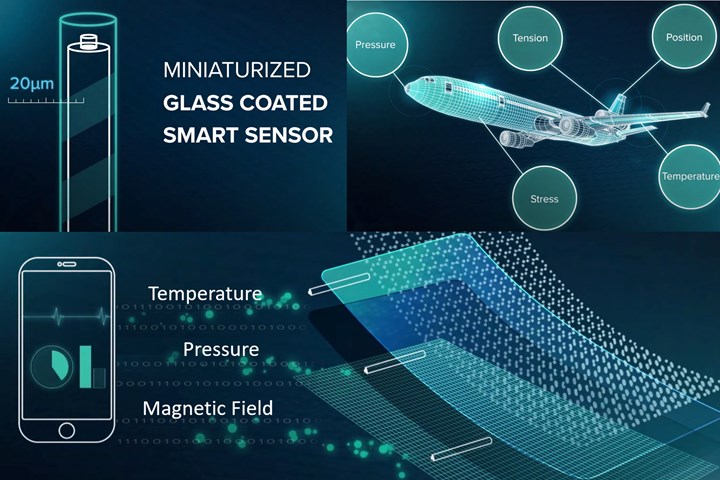
I have written about microwire sensors before (see “Measuring temperature inside composites and bondlines”). However, at that time, in 2018, I did not realize the long history of this technology, the wide breadth of published research on its development, nor the rapid rise of magnetic materials worldwide for a variety of measurement and sensing applications.
What I did understand was the disruptive possibilities of being able to measure, without contact, temperature from underneath 25 millimeters of carbon fiber-reinforced polymer (CFRP) inside a bondline or laminate during parts production and afterward, while the part is in service. Equally disruptive, was the potential to use a contactless microwire sensor instead of thermocouples to measure temperature and pressure inside a weldline during thermoplastic composite assembly. In fact, I was frustrated that more people in composites didn’t see the huge potential that I could see.
RVmagnetics (Košice, Slovakia), founded in 2015, has fully understood the potential of microwire technology for composites and beyond. “I have worked in basic and applied magnetism for 25 years, and started research with microwires in 2000,” says Dr. Rastislav Varga, RVmagnetics cofounder and professor at Pavol Jozef Šafárik University (UPJS, Košice, Slovakia). “We started with four persons and have now grown to 16, developing unique sensors for innovative leaders in a wide array of market sectors.”
Microwire sensors can sense temperature and pressure through a 2-millimeter-thick titanium skull impant or in a spinal cord … but they are ideal for composites.
“Because microwire is coated with glass, it is biocompatible,” notes Vladimír Marhefka, RVmagnetics chief marketing and strategy officer. “We are developing sensors to aid with medical implants, for example. One of the issues is that if something goes wrong with such implants, you don’t know what happened. We have developed sensors that can monitor temperature through a 2-millimeter-thick titanium skull implant. We can also continuously monitor temperature and pressure in the spinal cord. This has been demonstrated via testing in mice.” He adds that the microwire’s glass coating also protects against corrosion, noting, “Now we have introduced microwire sensors into concrete.”
“But microwire is ideal for composites,” says Varga. “Not only is it difficult to monitor the structural health of composite structures in service, we also see a need to monitor the processes used to make composites. Composites are typically thin, so it is very difficult to place sensors inside without creating defects or stress risers. For example, a strain gauge is very thin with an area of only 1 square centimeter. But even so, you produce a defect with these; the composite will break along the sensor. The advantage of microwire is that its diameter is similar to a human hair, so it does not decrease the performance of the composite. We have placed microwires inside of glass and carbon fiber composites and interrogated the sensors. We have always achieved successful results.”
The physics of amorphous and nanocrystalline microwires
Magnetic sensors offer numerous advantages due to their elevated sensitivity, reduced size and ability to sense without being powered through wired connections [1]. Note, the wireless interrogation devices are powered, but the sensors are not. Although studies dealing with magnetic microwires started in the 1970s, extensive research and progress has been made especially during the last decade [2]. Though there are many types of magnetic microwires, in general, they comprise a ferromagnetic metallic core, the most frequent elements being iron and cobalt, with a typical diameter of 0.5 to 90 micrometers (μm) and an insulating Pyrex glass coat with a thickness between 0.5 and 20 μm [1,2]. RVmagnetics microwires vary from 3 to 70 μm in diameter.
REFERENCES
[1] “Wireless stress sensor based on magnetic microwires” by Pilar Marín, published Nov. 29, 2017 by Intechopen.
[2] “Possible applications of magnetic microwires in aviation” by Katarína Draganová, Josef Blažek, Dušan Praslička and František Kmec, Department of Aviation Technical Studies, Faculty of Aeronautics, Technical University of Kosice, Slovakia. Fatigue of Aircraft Structures, Vol. 1, 2013.
[3] “Magnetostriction vs. Magnetoselastic Effect” by N. Lupu, European School on Magnetism, Cluj-Napoca, Romania. Sep. 9-18, 2007.
[4] “Novel applications of bistable magnetic microwires” by R. Sabol, P. Klein, T. Ryba, L. Hvizdos, R. Varga, M. Rovnak, I. Sulla, D. Mudronova, J. Galik, I. Polacek, J. Zivcak and R. Hudak. , Vol. 131, No. 4, 2017Acta Physica Polonica A.
[5] “Mechanical stress dependence of the switching field in amorphous microwires” by Rudolf Sabol, Marian Rovnak, Peter Klein, Manuel Vazquez and Rastislav Varga, IEEE Transactions on Magnetics, Vol. 51, No. 1, January 2015.
Fabrication
Diagram of microwire fabrication process (left), image from RVmagnetics production video (right). Photo Credit: (left) Fig. 2, “Possible applications of magnetic microwires in aviation”, (right) RVmagnetics production video.
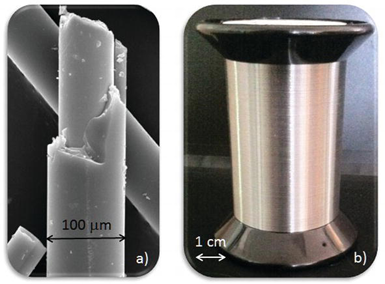
(a) SEM image of an amorphous Fe76Si9B10P5 microwire and (b) coil of microwire containing 2 grams of material. Photo Credit: Fig. 1, “Wireless stress sensor based on magnetic microwires”.
Glass-coated microwires are most commonly produced using the Taylor-Ulitovsky method, where several grams of a metal alloy with a specific chemical composition are placed into a glass tube. Using high-frequency induction heating, the metal is melted, which in turn melts the glass tube around it, trapping the metal inside. Both are drawn into a composite filament and rapidly quenched as they are wrapped onto a rotating spool. Continuous microwire up to 10 kilometers in length can be produced at a very low cost: 1 kilogram of alloy can produce 40,000 kilometers (~ Earth’s circumference at the Equator) of microwire at several hundred meters/minute.
This fabrication process also allows control of the microstructure and geometry (core diameter and coating thickness), which results in different mechanical, chemical and electrical properties. Cooling is a key process parameter. Another key factor is the chemical composition of the metallic core. Microwires commonly use amorphous metallic cores — for example Fe38.5Ni39Si7.5B15 or Fe76Si9B10P5 — but also nanocrystalline alloys like FeNiMoB, which give higher temperature capability, for example, up to 600°C. Microwire fabrication has been demonstrated to produce sensors with repeatable, reliable properties.
Magnetic properties, transmitting data
Glass-coated microwires commonly use soft magnetic materials — meaning they are easily magnetized and demagnetized — but also metals that exhibit magnetostriction, meaning they change their shape when subjected to a magnetic field. Magnetostriction results from the interaction between magnetization and strain of a magnetic material (magnetoelastic coupling) and it is an intrinsic material property. In the case of a microwire, its magnetostriction results from the specific alloy in its metal core and the final filament’s aspect ratio plus internal stress imparted during production.
Two characteristics enable magnetic microwires to be excellent sensors. First, their magnetostriction and anisotropy (long length and small diameter) make them extremely sensitive to small changes in mechanical stress. This sensitivity can then be translated into changes in their magnetization when subjected to an external magnetic field. This sensitivity can also be tailored. “In composites, we may want to measure from -50°C up to 150°C,” Varga explains, “while in the human body, we want the temperature range to be much narrower, and instead down to 0.01°C in sensitivity. Thus, we play with the chemistry of the microwire to fine-tune these properties.” The second characteristic is magnetic bistability.
Magnetic bistability, switching field and sensitivity
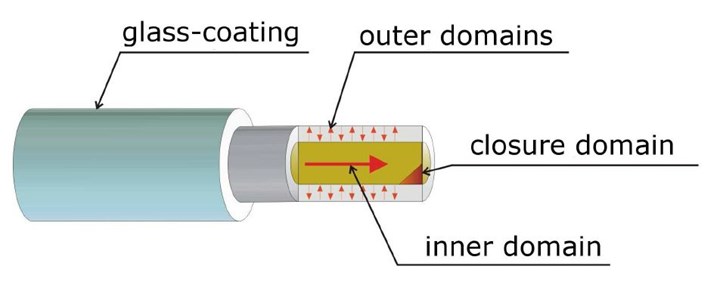

The domain structure of an amorphous glass-coated microwire with positive magnetostriction (top) and its bistable behavior illustrated by its hysteresis loop (bottom) of induced magnetic flux density (B) versus magnetizing force (H). Photo Credit: Fig. 1 and 2, reference [5] above.
Magnetic bistability is determined by a material’s magnetic domain structure. A magnetic domain is a region within a magnetic material in which the magnetization is in a uniform direction. Microwire metallic cores have a central axial magnetic domain surrounded by a radial domain (see figure, right). There are also closure domains at the ends of the microwire. This structure causes a bistable behavior — in other words, magnetization has only two states: +MS and−MS, where M S is the saturation (ultimate) magnetization. This magnetic bistability is not without limit; for example, it can be lost when the microwire length is shorter than the critical value determined by the diameter of its metal core and size of its closure domain [4,5]. This is why RVmagnetics works with its customers to design microwires for specific applications. Its sensors are typically 1 to 4 centimeters in length.
Switching between the two states, +MS and−MS, occurs through a single Barkhausen jump (sudden change in magnetic flux density or output as the applied magnetizing force is changed) at the value of the magnetic field called the switching field (HSW). This is illustrated in the hysteresis loop shown above. The switching field of bistable magnetic microwires is sensitive to external parameters including temperature, mechanical stress and magnetic field.
RVmagnetics uses a specially designed system that transforms the measured value (e.g., temperature or mechanical stress) into the switching time [4,5]. This allows RVmagnetics to distinguish between the contribution of the magnetic field and that of the value being measured, namely temperature or mechanical stress — an advantage versus measuring amplitude of the sensor signal. Moreover, the measurement of switching time instead of the switching field, HSW, drastically increases the sensitivity of microwire sensors. This has been demonstrated by Professor Varga and his co-authors in various technical papers describing experiments to assess the ability of microwires to measure changes in bending force, temperature and other parameters.
A microwire sensor — with a 45-µm diameter metallic core of Fe76Si9B10P5, 55-µm total diameter and 4-cm length — was bonded onto a CFRP laminate made from Sika Carbodur and subjected to a positive bending force. The resulting graph at right shows the variation in the time of the microwire’s switching field (y-axis) with positive bending. Photo Credit: Fig. 3, 4 and 6, reference [5] above.
Contactless sensing in composites
“If you use electrical sensors in composites,” notes Varga, “you must make a hole in the laminate to connect the wire to interrogate the sensor. Microwires fit in between the fibers in a laminate and you do not need to contact them to retrieve their data. We have compared samples of coupons with and without microwire, and there is no difference in mechanical properties.” This is also what AvPro (Norman, Okla., U.S.) reported for its microwire-based ThermoPulse sensors, as described in my 2018 article linked at the beginning of this blog. “In fact,” notes Varga, “you cannot find our sensors without a magnetic interrogator working under special conditions.”
“The problem with magnetic wires,” he continues, “is that they are sensitive to temperature and stress. At RVmagnetics, we have developed how to differentiate and distinguish between temperature, stress and magnetic effects. This is dependent on the microwire’s chemistry and also how you detect its signal. For example, if you have a magnet or mobile phone close to the microwire sensor, you will influence its signal.”
Photo Credit: RVmagnetics technology video
How close does the interrogator have to be to the microwire? Is it possible to go beyond 10 centimeters? In general, the issue is balancing distance and power, notes Varga. “If you need to be further away from the microwire, then you may need more power, but we try to keep the interrogation frequency below 10 kilohertz for safety reasons. We try to solve these issues through clever design of the microwire and keep the sensing system simple and cheap: just two coils, a couple of wires and a small amount of simple electronics.”
What resolution can microwire sensors provide? “The problem is how to process so many measurements,” says Varga. “We can place millions of sensors on a panel, sensing at 10 kilohertz, but this generates billions of data points per second. You don’t need all of this. In composites, our normal measurement rate is once every minute. For example, at 2-centimeters deep, we were measuring stress. Inside, we could see the fibers were sliding and that the resin was not cured properly. If you need to monitor stress throughout cure, you may need a few measurements per second.” Varga notes that RVmagnetics has developed cure monitoring. “We have developed the microwire chemical composition so that it can be cured with a composite up to 500°C.”
How many microwire sensors are required? “If you want to measure temperature distribution across a large panel, then many sensors are required, but a spinal cord implant can be measured using one sensor and one interrogator,” says Varga.
Different processes, parameters and cost
Is it possible to use microwire sensors in processes such as 3D printing? “We already have experience with placing microwire sensors inside of 3D printed parts,” says Varga, “either continuously or you stop and place the microwire precisely where you need a measurement. You can also measure temperature gradients inside the composite in each layer.”
How is it possible to continuously place a microwire sensor? “In GFRP rebar, we were able to place microwires without changing how it is made,” says Varga. “The wires are discontinuous but are placed automatically on a continuous basis as the rebar is produced. You have a coil with 1 kilometer of microwire and feed it into the rebar production equipment. It does not change the cost of the composite because it is a small amount of material and the cost is very low anyway. For example, one kilometer of microwire uses only one gram of iron. Even if you use platinum, by the time you divide one gram into hundreds of sensors, the price is very small.”
Compressive stress dependence of FeSiBP microwire switching field (red arrow shows microwire) embedded inside a block of concrete (left) and temperature dependence of the switching field in a nanocrystalline FeMoBCu microwire. Photo Credit: Fig. 2 and 4, reference [4] above.
What about welding of thermoplastic composites? “It is clear that using thermocouples to measure temperature in welding is a problem,” says Varga. “Induction welding depends on eddy currents, and these are reduced with something very thin in diameter such as a microwire. So, the effect of the induction welding equipment on the microwire is not the issue, but instead how to design the microwire and sensing to differentiate between temperature, stress and magnetic field, which we know how to do.”
He also notes that the unique properties of microwires lend a flexibility that is perhaps not anticipated. “For example, theoretically, you cannot put metal in a microwave oven. But we can use microwires inside a microwave. The issue is to have the correct positioning of the microwire within that specific electromagnetic field.”
“We have performed tests in our lab proving the low influence of induction welding on our microwire sensors,” Varga continues, “and are currently running tests with a manufacturer of induction-welded and -heated composites to further assess our technology on a larger, industrial scale.”
What about measuring vibration? “This is simply measuring stress at a higher frequency,” says Varga.
Resin flow? “We measure the change of pressure and strain,” he explains. “For example, envision the hairs on your arm. As air passes over, you can feel the change. Now think of these hairs as microwires — when placed on a surface, they will bend during flow.”
Electric current? “When you have a conductor, it produces an electromagnetic field,” says Varga. “So, we just measure that. Our microwire usually measures two parameters simultaneously — either temperature/electromagnetic field or stress/electromagnetic field. We are able to measure all three by placing two microwires together, and we use the same interrogation process to monitor the output.”
RVmagnetics has also developed PC-based software to process the digital data output. “We work with the customers to make sure the system includes what is needed per each application,” he explains.
Marhefka notes that microwires can be used to replace existing sensors. “We can compete on price, sensitivity, size or simplicity of measurement, and can also enable novel sensing capabilities.” RVmagnetics’ emphasis, however, is on problems that have not yet been solved. “Composite companies haven’t had contactless solutions to measure temperature and stress inside of a composite, thermoplastic weld or adhesive bondline,” he observes, “but now they do.”
Related Content
Infinite Composites: Type V tanks for space, hydrogen, automotive and more
After a decade of proving its linerless, weight-saving composite tanks with NASA and more than 30 aerospace companies, this CryoSphere pioneer is scaling for growth in commercial space and sustainable transportation on Earth.
Read MoreAchieving composites innovation through collaboration
Stephen Heinz, vice president of R&I for Syensqo delivered an inspirational keynote at SAMPE 2024, highlighting the significant role of composite materials in emerging technologies and encouraging broader collaboration within the manufacturing community.
Read MoreJEC World 2023 highlights: Recyclable resins, renewable energy solutions, award-winning automotive
CW technical editor Hannah Mason recaps some of the technology on display at JEC World, including natural, bio-based or recyclable materials solutions, innovative automotive and renewable energy components and more.
Read MoreHexagon Purus opens new U.S. facility to manufacture composite hydrogen tanks
CW attends the opening of Westminster, Maryland, site and shares the company’s history, vision and leading role in H2 storage systems.
Read MoreRead Next
Measuring temperature inside composites and bondlines
ThermoPulse sensors offer Industry 4.0 temperature measurement and digital cure cycle management for bonded composite repairs, laminates and more.
Read MoreSensors: Data for next-gen composites manufacturing
In the quest for sustainability, sensors are reducing cycle times, energy use and waste, automating closed-loop process control and increasing knowledge to open new possibilities for intelligent manufacturing and structures.
Read MorePlant tour: Daher Shap’in TechCenter and composites production plant, Saint-Aignan-de-Grandlieu, France
Co-located R&D and production advance OOA thermosets, thermoplastics, welding, recycling and digital technologies for faster processing and certification of lighter, more sustainable composites.
Read More