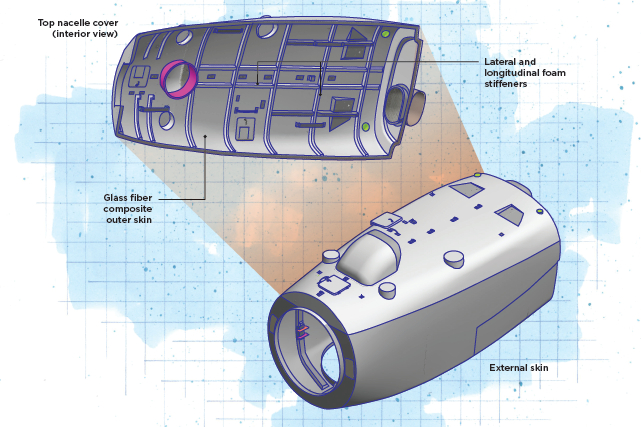
Glass fiber-infused nacelle cover drawing. Illustrated by Susan Kraus.
- Optimized three-part design reduces foam core usage with strategically placed foam core stiffeners.
- Four-millimeter-thick outer skin manufactured from glass fiber and custom epoxy/polyester resin.
- In-situ molded stiffeners decrease overall cycle times.
Global wind energy installations surged in 2020, with 93 gigawatts (GW) of new capacity added, bringing the world total to 743 GW wind power capacity, according to the Global Wind Energy Council’s (GWEC, Brussels, Belgium) 2021 report. However, to meet global climate change goals of net-zero carbon emissions by 2050, the GWEC says that wind power needs to be installed three times faster, with up to 280 GW new capacity installed annually by 2030.
To meet rising demand for renewable energy, larger and more powerful wind turbines, with longer turbine blades, continue to be developed and installed. In addition, to compete with low-cost fossil fuel energy, wind energy also needs to be generated at a low levelized cost of energy (LCOE), which is an estimated cost of both building and operating a generator. Wind turbine manufacturers are challenged to not only increase production of more powerful turbines, but to help wind farms lower LCOE by producing wind turbine components more cost-efficiently, optimizing equipment costs.
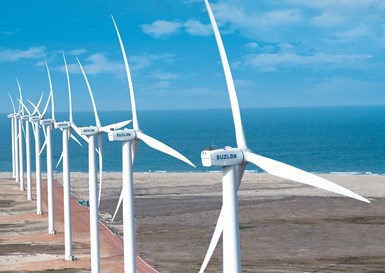
Resin-infused, glass fiber composite nacelle covers. As the demand for larger and more powerful wind turbines continues to rise, wind turbine manufacturers like Suzlon Group continue to investigate ways to optimize their structures and processes. A novel hat stiffener design enabled Suzlon to reduce cycle times and material costs on its nacelle covers. Photo Credit, all images: Suzlon Group
Wind turbine blades, as increasingly large, all-composite structures, are understandably a target for cost reduction, but they are not the only composite components on wind turbines that may require some reengineering as the industry steps up to meet demands for renewable electricity with a low LCOE. The nacelle cover, the large protective and structural housing for the wind turbine generator and other electronics, is also a heavy user of composites.
Nacelle covers are often manufactured via resin-infused glass fiber composites to meet size, complex geometric and weight requirements. Regulated by standards and guidelines issued by certifying bodies like DNV GL (Høvik, Norway), the International Electrotechnical Commission (IEC, Geneva, Switzerland) and TÜV (Cologne, Germany), nacelle covers are critical structural components that protect the turbine machinery against external hazards like precipitation, dust, UV radiation and lightning strikes, as well as provide an inlet and outlet for airflow within the wind turbine generator, withstand the wind forces experienced by the blades and heat produced by the nacelle gearbox and other machinery and provide a safe working platform for service personnel.
Suzlon Group (Pune, India) is a wind turbine manufacturer and renewable energy solutions provider that reports more than 18.8 GW of installed wind capacity globally to date. Some of its research has focused on ways to optimize designs and manufacturing processes to help the company meet global and domestic market demands for more turbines at lower LCOEs. According to Fatehali Alchiya, president of design and product engineering at Suzlon Group, these needs led the company to explore optimization and innovation potential for every component of its turbines, with the aim of reducing total component costs by means of material consumption and/or manufacturing costs.
During this evaluation, one of the components that Suzlon Group re-evaluated and redesigned was its glass fiber composite, 10-meter-long x 4-meter-wide x 4-meter-tall nacelle cover for its 2.1-megawatt (MW) onshore wind turbines.
From sandwich panels to hat stiffeners

Previous sandwich construction. Originally, the 24-millimeter-thick nacelle cover comprised an internal and external skin cored with foam.
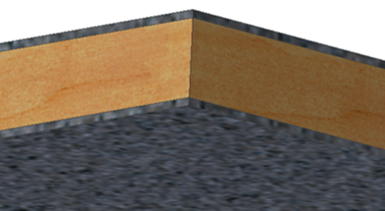
From conceptualization to building the first prototype, the development process took approximately 11 months beginning in April 2016.
For ease of assembly, the overall nacelle enclosure is manufactured in three parts — upper, lower left and lower right — using a resin infusion molding process at Suzlon’s manufacturing facility in Daman, India.
The original configuration of the upper cover section of the enclosure was a 24-millimeter-thick, resin-infused sandwich panel comprising inner and outer glass fiber skins cored with foam. Suzlon wanted to develop an alternative cover design that reduced material costs through a reduction in foam usage, while still maintaining the necessary stiffness, load capability and compression and tensile strength — especially important because the nacelle cover functions not only to protect the turbine’s machinery against environmental forces and wind, but to protect and bear the weight of humans and maintenance equipment.
The optimized nacelle cover design would need to be the same dimensions as the previous version, with the same mounting brackets, hardware and additional interfaces built into the geometry. In addition to reduced material usage and associated costs, the cover also needed to be manufactured in a relatively fast, cost-effective, repeatable process to accommodate ramped-up production volumes.

Optimized hat stiffener design. The new design minimizes foam core use while maximizing stiffness, incorporating longitudinal and lateral foam core hat stiffeners in between glass fiber skins. The top image shows a cut-out model of the nacelle’s upper cover. The cross section (bottom image) shows the “hat” stiffener shape.

First, the team’s structural analyst generated parametric finite element analysis (FEA) models using ANSYS (Canonsburg, Pa., U.S.) Workbench software and performed experimental design studies on 240 design combinations to establish the optimum design parameters. Based on the study results, the options were narrowed down to the four top potential designs, which were further evaluated for manufacturing feasibility. The final design concept was prepared as a CAD model using Autodesk (San Rafael, Calif., U.S.) Inventor.
Ultimately, through a combination of virtual testing and laminate coupon tests, the researchers settled on a stiffener-based design that provided more than twice the stiffness of the original sandwich construction, with significantly less use of high-cost foam core.
The optimized, hat stiffener design concept comprises a 4-millimeter-thick outer glass fiber composite skin, reinforced by the placement of dedicated, load-carrying “hat” shaped stiffeners constructed from foam core at specific, high-load areas of the part. An additional 4-millimeter skin is placed over the stiffeners. On the final design, the cover includes four longitudinal and 13 lateral hat stiffeners.
Optimizing resin, manufacture and assembly processes
Each section is constructed from E-glass fabric, and the foam core can be made from PVC, SAN, PU or PET. To protect against lightning strikes, a conductive mesh is layered between the fabrics per Suzlon’s patented design. For the resin, N. Muthukrishan, senior general manager of structural design at Suzlon Group, says that a custom epoxy and polyester formulation was developed in partnership with Suzlon’s resin supplier, because none of the options on the market met the company’s specific requirements for gel time, wetting time and time to peak temperature.

In-situ molding. The foam core hat stiffeners are placed strategically within the part prior to resin infusion (top) and molded in-situ under vacuum (bottom). Manufacture of the upper cover is pictured here.

To accommodate higher targeted production volumes, various options for integrating the foam core hat stiffeners with the skin were investigated. Muthukrishnan says Suzlon considered bonding prefabricated stiffeners onto the main skin as a post-molding activity, similar to the way boat hulls are manufactured. Co-lamination and mechanical fastening were also considered as options. However, the option that showed the fastest cycle times and greatest efficiency was in-situ molding of the stiffeners to the skin.
To build the cover, cut fabrics are placed into composite molds that are developed in-house by Suzlon. Foam core hat stiffeners are CNC machined and placed in pre-determined positions on the inner surface of the main skin, followed by another layer of glass fabric placed over the foam. The configuration is vacuum-bagged and then resin infused. One challenge to the new design was how to ensure even resin flow around the stiffeners. Resin feed lines needed to be positioned carefully for even wetting across the component, and Suzlon worked with the resin supplier to provide a resin with a longer gel time to allow for the resin to take longer to spread in some areas of the part than in others (infusion is said to take 60 to 120 minutes per cover section, based on part complexity and size).
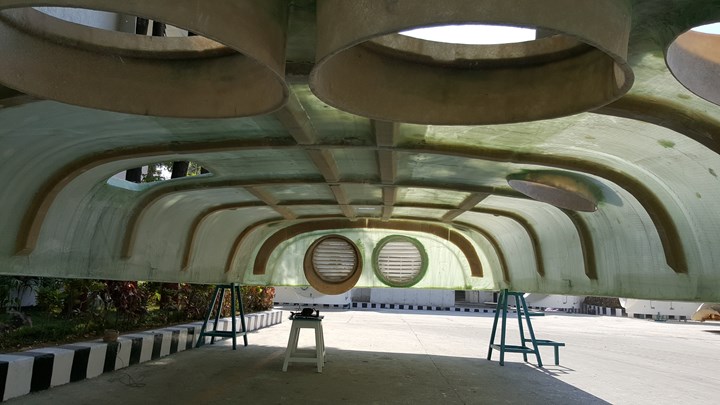
The finished top cover. The three pieces of the final nacelle enclosure, including the pictured upper cover shown after molding and trimming, are mechanically fastened together at Suzlon’s facility. The large holes shown here in the top cover are to enable ventilation of the nacelle.
After infusion, the components are cured via a one-hour pre-cure at room temperature to remove entrapped air, followed by a seven-hour cure at elevated temperature via external heaters in a controlled environment. The three finished nacelle enclosure components are assembled into the final structure using mechanical fasteners. The process of in-situ molding multiple stiffeners under vacuum won Suzlon an ICERP-JEC Innovation Award in 2019.
Overall, Suzlon reports that the in-situ molding process resulted in a 10% reduction in cycle time and 35% reduction in foam core use compared to the production process of sandwich panels, cost savings which enable Suzlon to produce these parts faster and more cost-effectively. To help wind farms achieve LCOE goals, according to Alchiya, these savings also result in an overall more affordable energy system for customers as well: “This optimization is considered to be one of the key contributors towards affordable cost of energy in wind turbine manufacturing,” he says.
The optimized turbine enclosure was first installed on Suzlon 2.1-MW turbines in fiscal year 2017-2018, and have been installed on more than 250 turbines to date and counting.
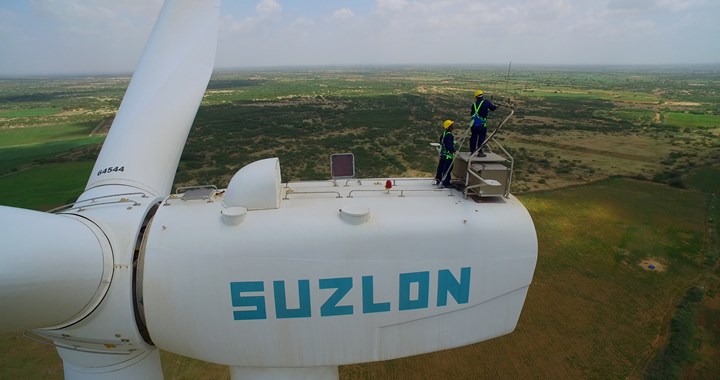
More than 250 installed to date. Suzlon continues to install 2.1-MW turbines with its optimized nacelle covers. One key design consideration was stiffness, both to protect the inside turbine from weather and other hazards, and to enable a safe surface for maintenance staff.
Related Content
One-shot manufacture of 3D knitted hybrid thermoplastic composite structures
MAPICC 3D project replaces steel seat support in heavy-duty vehicle with a 3D knitted composite made from thermoplastic hybrid yarns comprising the matrix and reinforcing components.
Read MoreProtecting EV motors more efficiently
Motors for electric vehicles are expected to benefit from Trelleborg’s thermoplastic composite rotor sleeve design, which advances materials and processes to produce a lightweight, energy-efficient component.
Read MoreASCEND program update: Designing next-gen, high-rate auto and aerospace composites
GKN Aerospace, McLaren Automotive and U.K.-based partners share goals and progress aiming at high-rate, Industry 4.0-enabled, sustainable materials and processes.
Read MoreOptimizing a thermoplastic composite helicopter door hinge
9T Labs used Additive Fusion Technology to iterate CFRTP designs, fully exploit continuous fiber printing and outperform stainless steel and black metal designs in failure load and weight.
Read MoreRead Next
Hydroelectric turbine blade design propelled by composites
Glass fiber composites power the development of a modular, spiral-shaped hydroelectric micro turbine blade for low-cost, high-efficiency renewable energy generation.
Read MoreReimagining wind blade design
Wind turbine blade architecture is so well established that it’s difficult to imagine there might be a better alternative. ACT Blade’s skeleton-based design is a step in that direction.
Read MorePlant tour: Teijin Carbon America Inc., Greenwood, S.C., U.S.
In 2018, Teijin broke ground on a facility that is reportedly the largest capacity carbon fiber line currently in existence. The line has been fully functional for nearly two years and has plenty of room for expansion.
Read More