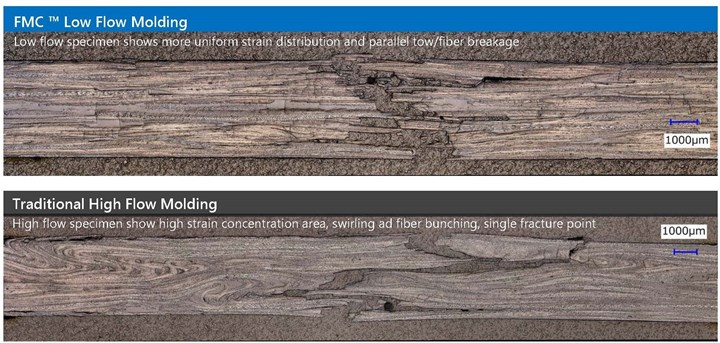
A key element of Mitsubishi’s new forged molding compound (FMC) carbon fiber-reinforced SMC is the use of low-flow (but not low-pressure) compression molding, which helps preserve isotropic fiber orientation/distribution in the material to yield parts with high complexity and high mechanical properties for use in structural applications (upper image; cross-flow direction). Using FMC in conventional high-flow compression molding (lower image; cross-flow direction) destroys the isotropic properties and can lead to property loss. Photo credit, all images: Mitsubishi Chemical Corp.
Sheet molding compound (SMC) is a workhorse composite — in the form of a tough, thermosetting, B-staged, compression moldable sheet-form molding compound — used commercially since the early 1960s. However, as compounders extend technology to more novel resins, reinforcement types and formats, SMC is being reinvented. With new kinds of SMC come new application opportunities, as with a new product developed by Mitsubishi Chemical Corp. (Tokyo, Japan) with engineering support from Gemini Composites LLC (Seattle, Wash., U.S.), which Mitsubishi acquired in 2017. Called forged molding compound (FMC), the material is an advanced SMC reinforced with chopped carbon fiber and developed for structural applications with fairly complex designs using a compression molding variant.
Highly moldable, isotropic and better performing
FMC edges closer to prepreg performance while retaining more of SMC’s processability and affordability. It combines industrial-grade carbon fiber tows, which are chopped and split into smaller bundles, then impregnated with vinyl ester (VE) or epoxy matrices. By splitting larger tows into finer bundles, it’s possible to achieve materials with better and more uniform mechanical performance at lower cost than starting with aerospace-grade tows. Cure occurs in 40-120 seconds at 130-150°C.
“While there are many different continuous carbon fiber composites, they have limited application,” explains Koichi Akiyama, president, Gemini Composites and one of the developers of FMC. “What we wanted to create was a high-performance, chopped carbon fiber material that could be used in a much wider variety of applications and formed on commonly available equipment. FMC technology really consists of three factors. It involves an advanced material, an optimized molding process and dedicated design. Together, these factors enable FMC to replace lightweight metals in structural applications at significantly lower weight.”
Foundational research
Interestingly, technologies that would prove key to FMC development began as separate research projects around 2015. Initially, the team’s focus was on increasing the performance of Mitsubishi’s own 3K and 15K chopped carbon fiber SMC (CF-SMC) rather than developing a new material. The goal was to produce a true isotropic composite with chopped fibers in complex shapes that offered mechanical performance closer to prepreg.
“Nothing was easy about trying to find ways to use chopped carbon fiber composites in structural applications with complex geometry at an affordable cost,” recalls Akiyama. “However, we were convinced that if we could find the right combination of material, process and design, then what had previously been impossible would become possible.”
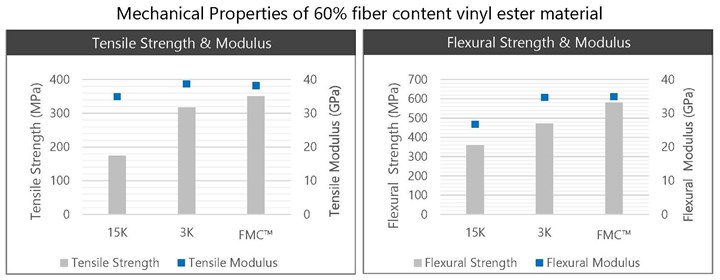
FMC takes advantage of a well-established phenomenon in the composites industry that smaller/finer carbon fiber tows yield materials with better fiber wetout/bonding and parts with better properties when compared to traditional SMC. Even though FMC starts with industrial-grade carbon tows, by splitting and then chopping them into fine tow bundles, FMC achieves better performance than even 3K CF-SMC in molded parts.
One focus was on formulation of high-performance epoxy resin and sizing systems to support robust mechanical properties and short molding cycles necessary for high-volume, cost-sensitive end markets like automotive. Another goal was to develop a method to affordably deploy small-tow count carbon fiber.
“It’s well established in the industry that smaller/finer carbon fiber bundles yield higher properties, but such fiber is expensive owing to manufacturing challenges and the resulting yield,” Akiyama explains. “That led us to start by splitting larger tows. Interestingly, tests show we’ve achieved higher molded properties with FMC than with 3K CF-SMC.”
A major challenge was to achieve isotropic fiber distribution between X and Y axes by developing a tightly controlled compounding process that eliminated the inherent machine-direction bias characteristic of all chopped fiber SMC. This bias leads to a higher coefficient of variation (CoV) of mechanical properties — a feature exacerbated by conventional high-flow compression molding.
“To achieve isotropic fiber distribution in a molded part, the starting material must have an isotropic fiber distribution,” explains Akiyama. “We had a clear vision of what the ideal shape, size and distribution of chopped fibers in the material needed to be. The big challenge was how to achieve this.”
By 2017, the team had developed the split-tow carbon fiber and high-performance epoxy resin system. Next, they finalized work on a special chopping unit, which not only produced fine fiber bundles (<3K tows) quickly and controllably, but disbursed/distributed those bundles isotropically across the sheet during SMC compounding.
“Split-tow carbon fiber does break up into smaller bundles during chopping, but those bundles weren’t small enough to provide the level of property enhancement we sought,” continues Akiyama. “We developed proprietary equipment and a process to break bundles up further and achieve the type of fiber distribution we believed would give us both excellent properties and good moldability.” Because FMC doesn’t rely on flow in the tool to help mix material, impregnation and compounding steps have to be carefully controlled to ensure very low variation in mechanical properties.
Low-flow, not low-pressure
Development soon accelerated thanks to opportunities to use FMC in primary body structures. The main challenge, as mentioned above, was how to mold it without losing those isotropic properties. Further testing indicated that if FMC was allowed to flow during compression molding like traditional SMC, it not only would lose isotropic fiber alignment, but excessive flow would produce defects and property loss. Fortunately, Gemini Composites, which had been developing low-flow molding for structural CF-SMC, proved a perfect fit for FMC. It also made Gemini an ideal acquisition target for Mitsubishi. Since then, Gemini has led FMC design and process development to ensure isotropic properties aren’t lost during processing.
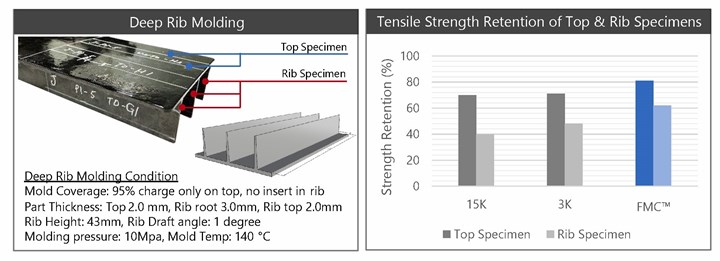
Even though FMC is capable of penetrating deeply into tall ribs, it does a better job of preserving tensile strength between base and tip than either 15K or 3K CF-SMC.
With FMC, material flow in the tool must be limited, which requires a different approach to mold design, part design and processing. For example, with straight vertical walls, it’s difficult to charge enough material on the feature. With conventional SMC, such walls are commonly filled by flowing material from another area of the part, inadvertently making the material (and resultant part) more anisotropic owing to greater fiber movement. To fill vertical walls with FMC, Akiyama says ingenuity and experience are needed to not only fill the geometry but also compensate for negative effects resulting from the inevitable material flow — a far more complex approach.
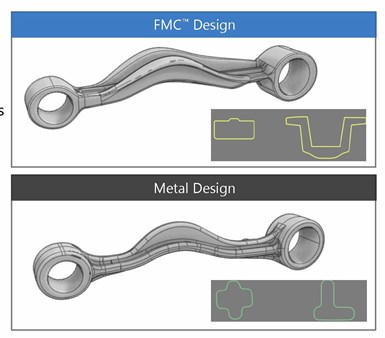
An early FMC demonstrator was an automotive suspension component ~362 x 63 x 70 mm and 3-13 mm thick.
“We can design with some angles if there is acceptable space in the part,” explains Akiyama. “And we can even use a straight wall if that is essential to the design. However, we would modify geometry in another area of the part to minimize flow up the vertical wall. We could also use special mold features like slides, or split the part to charge enough material to fill the wall. Therefore, it’s very important for the part designer, toolmaker and the Gemini team to work closely so both part and mold are low-flow friendly.”
It’s important to clarify that while material should not flow much — with layups designed to cover 80-95% of the tool before press closure to purposely leave little room so as to restrict flow — the process is neither no-flow, nor low-pressure molding. This is in comparison to average tool coverage with traditional SMC which, while dependent on part complexity, surface finish, etc., typically ranges from 50-60% and can be as broad as 30-70%. Additionally, the usual SMC molding pressures of 5-12 MPa are also used in FMC. Fulfilling one program goal, properly designed parts can fill complex geometry like ribs. Reportedly, FMC retains 85% and 66% of nominal tensile strength at, respectively, the top and bottom of deep ribs. Akiyama says it fills complex geometry better than 3K CF-SMC.
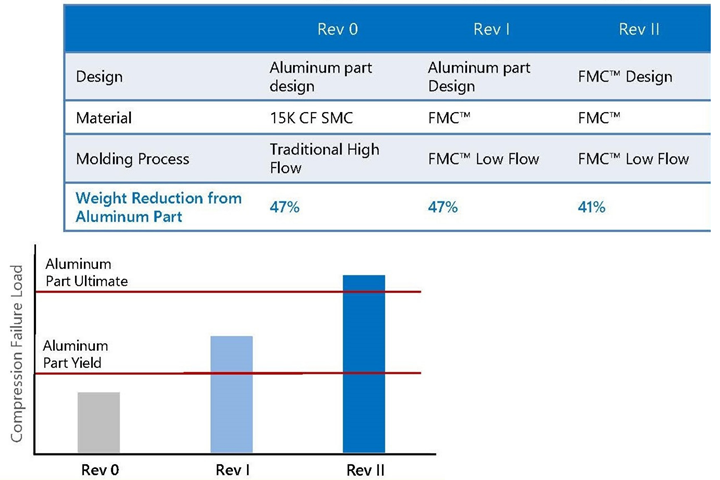
The benchmark cast aluminum part was first directly converted to 15K CF-SMC (Rev 0) using the metal design and high-flow molding. This reduced mass 47% vs. the benchmark, but at lower compression performance. Next, the metal design was minimally modified to be moldable in FMC with low-flow molding (Rev I). This design achieved the same 47% mass reduction, but at somewhat higher compression performance. Finally, the part was redesigned specifically to take advantage of FMC material and low-flow molding (Rev II). This time, mass was 41% lower but compression performance was much higher than the benchmark.
Given the large mold coverage of a charge of FMC, it’s useful to lay up near net-shape charges in a preform jig like conventional prepreg or SMC. This helps balance the shape and weight of the charge pattern and speeds manual or robotic loading of the press.
New opportunities
“With this technology, we’ve created a more homogenous structure that provides excellent mechanical properties and dimensional stability at much-lower CoV versus traditional SMC,” Akiyama continues. “There are more fiber layers in the same thickness of material than are seen with 3K CF-SMC, yet there are also fewer resin-rich areas owing to the excellent wetout we achieve. This enables us to provide better properties more economically.”
He notes that FMC technology actually makes simulation work easier and more accurate than with conventional SMC, where molded parts often have lower-than-predicted properties after material flow and fiber orientation changes. That flow can also cause defects, which lead to failure at lower loads. “With FMC technology, we can eliminate most of the trial and error usually needed to develop structural applications,” Akiyama adds.
While raw material prices will likely be higher for FMC than aluminum, short molding cycles, light parts and near-net shape designs reduce material usage and secondary finishing, which should help make FMC parts roughly cost-comparable to aluminum parts. “Replacing machined metal parts is an excellent application for FMC,” Akiyama adds. “Since we can make a mold for the cost of machining a few metal parts, if more than a handful of parts are needed, we will be competitive.”
Not yet ready for commercial production, which should begin later this year, Mitsubishi is sampling VE- and epoxy-based resin systems to interested customers, while Gemini provides engineering support. Epoxy formulations permit room-temperature storage for up to two weeks, unlike conventional epoxy prepreg, which requires cold storage. The first commercial applications are likely a few years away. Although the company can theoretically cut its split-tow fibers to any length, they initially will be offered in standard 13, 25 and 50 millimeters, with fiber-volume fractions of 40-65%. Grades with better flame/smoke/toxicity (FST) properties and higher glass transition temperatures (Tg) are said to be in development. Targeted automotive applications include components for large body structures, suspension, powertrain, crash box and EV battery enclosures. Other industries considering FMC include aerospace/defense and industrial.
Related Content
Graphene-enhanced SMC boosts molded component properties
CAMX 2023: Commercially sold GrapheneBlack SMC from NanoXplore increases part strength, stiffness and provides other benefits for transportation, renewable energy, energy storage and industrial markets.
Read MoreCAMX 2022 exhibit preview: Cimbar Performance Minerals
Cimbar’s halogen-free ATH solution for thermoset applications reduces resin costs in formulation, provides better wetout and faster line speeds and improves mold flow.
Read MoreMaterials & Processes: Resin matrices for composites
The matrix binds the fiber reinforcement, gives the composite component its shape and determines its surface quality. A composite matrix may be a polymer, ceramic, metal or carbon. Here’s a guide to selection.
Read MoreImproving carbon fiber SMC simulation for aerospace parts
Simutence and Engenuity demonstrate a virtual process chain enabling evaluation of process-induced fiber orientations for improved structural simulation and failure load prediction of a composite wing rib.
Read MoreRead Next
All hail SMC
Sheet molding compound (SMC) is no stranger to the composites industry, but the last few years have seen a renaissance for this workhorse material as it is embraced anew.
Read MoreFrom the CW Archives: The tale of the thermoplastic cryotank
In 2006, guest columnist Bob Hartunian related the story of his efforts two decades prior, while at McDonnell Douglas, to develop a thermoplastic composite crytank for hydrogen storage. He learned a lot of lessons.
Read MoreCW’s 2024 Top Shops survey offers new approach to benchmarking
Respondents that complete the survey by April 30, 2024, have the chance to be recognized as an honoree.
Read More