A400M cargo door: Out of the autoclave
This structural military airframe part is the largest made to date via the vacuum-assisted resin infusion process.
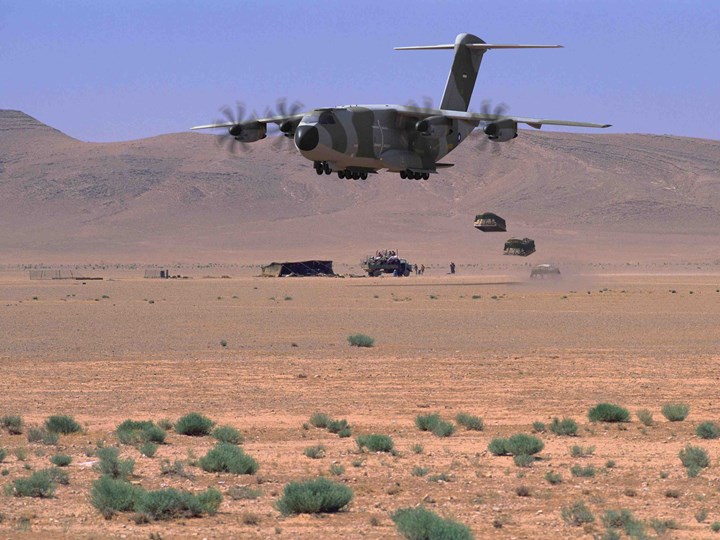
The A400M’s unusual split cargo door configuration permits tactical maneuvers such as equipment/supply drops, without the necessity to land and take off.
Photo Credit: Airbus Military
Airlift capability and supply portability are key elements in any country’s military and emergency preparedness. Until now, Boeing’s C-17 Globemaster and Lockheed Martin’s C-130J Hercules, introduced decades ago, have made up the bulk of the world’s roughly 2,300 aging military transports, and many are due for refurbishment. The former business unit of Airbus, Airbus Military, hoped to capture a chunk of this strategic market with its A400M cargo plane.
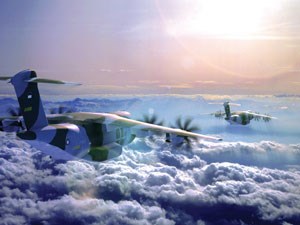
Artist’s conception shows aft end of cargo hold, where the two-part cargo door forms the smooth, upswept underbelly of the aft fuselage. Photo Credit: Airbus Military.
This airlifter, slightly larger than the C-130J but smaller than the C-17, is composites-intensive — the design and manufacture of the A400M’s composite wing spars, which will support the world’s largest turboprop aircraft engines, were described in a previous issue of HPC. The aircraft’s vertical and horizontal tailplane, produced in Spain, is also a large carbon fiber/epoxy component.
In January of this year, the first A400M upper cargo door — the largest structural composite aircraft component produced to date using the vacuum-assisted resin infusion process — was delivered by the European Aeronautic Defence and Space Co. (EADS) Military Air Systems Business Unit (Augsburg, Germany) to Airbus for the first fuselage assembly. EADS Augsburg is the largest external supplier for the Airbus family and through its Center of Competence has helped de-sign and promote innovative materials and technologies for aerostructures. One of the cargo doors was recently on display at the JEC Composites Show in Paris, France. The door represents a significant breakthrough for low-cost, out-of-autoclave processing in a highly demanding structural application.
Design for lower cost
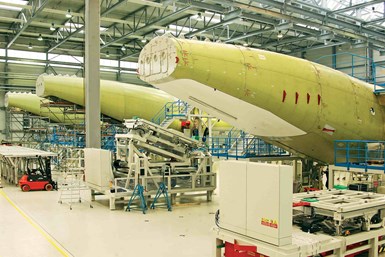
Three A400M fuselage sections stand ready for door installation, with a composite upper section already in place on the nearest unit.Photo Credit: Airbus Military.
The A400M, with its four turboprop engines and high wing, is designed with cargo doors that open at the rear of the fuselage. The 7m/23-ft long by 4m/13-ft wide all-composite upper cargo door, described in this article, swings upward and inward while a lower cargo door, equipped with a metal ramp, simultaneously rotates outward and down to allow vehicles and cargo to be loaded or unloaded. When closed, the two doors form the smooth rear fuselage underbelly.
The geometrically complex upper door was designed for the lowest possible weight, says an EADS Augsburg design engineer, to reduce loads on the door mechanism and to help meet the overall A400M weight target. Less weight in the airframe means larger payloads can be hauled. In addition, part stiffness had to be maximized because the door is located within the pressurized portion of the fuselage, subject to significant fatigue loading during pressurization cycles.
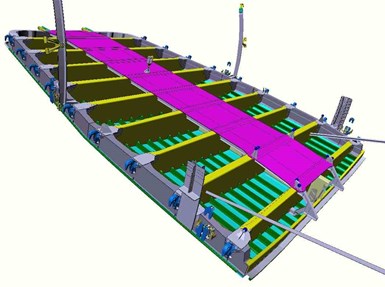
Step 1: CAD drawing shows overall part design: Outer plate is blue-green in color, integral stiffening stringers are dark green and the crossbeams are shown in yellow. The smaller, partial inner door is shown in pink. Note the endwalls around the perimeter of the part where the large hooks attach for sealing the door in flight. Photo Credit: EADS Military Air Systems, Augsburg.
EADS engineers used finite element analysis (FEA) software to design the door for the anticipated loads. They also opted to forego the autoclave and make the door using EADS’ patented infusion technology, dubbed VAP (vacuum-assisted process), to minimize production costs. As do many other infusion processes now in use within the composites industry, VAP employs a vacuum to facilitate epoxy resin infusion of dry carbon fiber reinforcements under a vacuum bag. The part then is cured in an oven rather than in an autoclave process.
The door comprises several elements. The largest, the outer skin, is a slightly domed and tapered rectangle made of a solid, uncored carbon/epoxy laminate. The skin’s inner surface is stiffened with 16 stringers oriented parallel to the fuselage’s long axis. A separate edge wall, roughly 203 mm/8 inches high, forms the perimeter of the part. Nine beefy crossbeams run perpendicular to the stringers and are attached to the skins with metallic fasteners. A narrow inner skin (see Steps 1 & 7, at right) covers the central portion of the door’s inside surface and functions as a partial inner for additional rigidity. It is also stiffened with several small stringers. A metal hinge bolted to the outer skin attaches the door to the fuselage, and large metal hooks affixed to the edge wall are used to hold the door in place during flight.
A key element of the design and VAP process is the fact that the stiffening stringers are integral and cocured with the door’s outer and inner skins, saving considerable production time and eliminating thousands of fasteners. The design team was able to dispense with roughly 3,000 metallic rivets that would have been required to attach the stringers to the outer skin, saving fastener weight and also considerable cost.
No autoclave needed
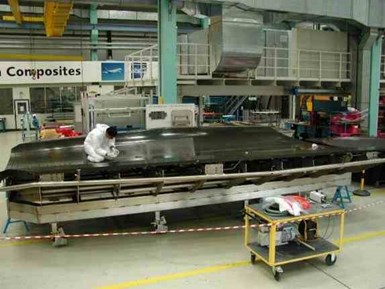
Step 2: A technician lays up the outer plate skin in a female composite tool, using dry multiaxial and unidirectional carbon fiber reinforcements. Photo Credit: EADS Military Air Systems, Augsburg.
Door fabrication begins with layup of the outer skin on a female carbon fiber/epoxy composite tool, designed and made in-house by EADS. While the company does not discuss the exact number and orientation of skin plies, a combination of dry carbon fiber multiaxial reinforcements supplied by SAERTEX (Saerbeck, Germany) in 0/90° and ±45° styles, as well as dry uniaxial fabric supplied by Hexcel (Dublin, Calif.), make up the laminate. The innermost ply (see Step 3) has additional buildups in the areas that will be contacted by stringers and crossbeams.
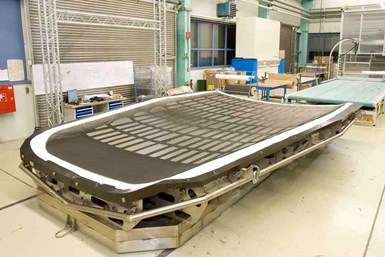
Step 3: The completed outer plate layup. Note the pattern of buildups at the locations of the stiffening stringers and crossbeams. Photo Credit: EADS Military Air Systems, Augsburg.
Augsburg engineers refer to the integral stiffening stringers as an “L-Z” design, essentially shaped like the capital letter Z with a vertical web, superimposed over a backward-facing capital letter L. Stringers are about 65 mm/2.5 inches high with a 50-mm/2-inch wide lower flange. Multiple plies in the top flange of the stringer drop off to about half the number in the vertical web;
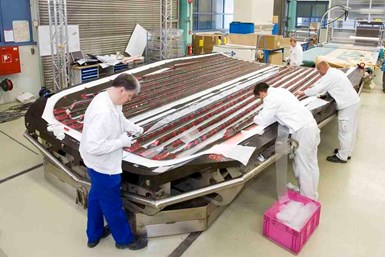
Step 4: Stringer performs are placed on the plate and bagged with GORE-TEX membrane. A series of tapes hold the performs and their associated tooling together during the bagging and infusion processes.Photo Credit: EADS Military Air Systems, Augsburg.
the web plies then split to form the horizontal lower flange. A combination of the SAERTEX 0/90° and ±45° multiaxial dry reinforcements and the unidirectional material from Hexcel make up the stringers. To hold the dry stringer reinforcements in place during preforming, Augsburg reports that some of the reinforcements are supplied with a thermoplastic polyamide veil or tissue on the top surface; when heated, the thermoplastic veil melts and becomes tacky, thus holding the multiple plies together.
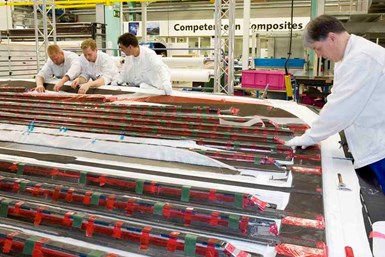
Step 5: A closeup of the bagging process, showing the stringers in place on the plate. Photo Credit: EADS Military Air Systems, Augsburg.
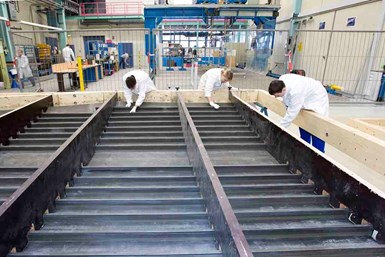
Step 6: After skin and stringer cure is complete, the partially completed part is readied for nondestructive inspection in a temporary wooden holding jig. Photo Credit: EADS Military Air Systems, Augsburg.
Technicians preform the stringers on an inexpensive wooden template by stacking the multiaxial strips and then heating them with a small heat gun to melt the thermoplastic and thus bond the plies together. Short ply buildups on the top flange are made with the unidirectional material; the 0/90° and ±45° material forms the continuous plies through the web. A triangular “noodle” or filler made with small-diameter carbon fiber braid is inserted into the void formed where the plies split to create the flat lower flange.
The preformed stringers are then placed on the layed up skin, over the buildups. Long, carbon fiber/epoxy mandrels are placed on both sides of each stringer to hold the preforms in the correct shape and to provide consolidation pressure during cure. Then, each stringer and its mandrels are covered with GORE-TEX gas-permeable membrane material supplied by W.L. Gore & Assoc. (Newark, Del.).
When all the stringers are in place and bagged, technicians then bag the entire door — the outer skin part and the stringers — with standard breather material and bagging film and prepare for the infusion. The resin is RTM-6 one-part epoxy, manufactured by Hexcel. This well-known infusion resin was reportedly easy to process, offering good hot/wet performance to 180°C/350°F, and it has a processing window of more than two hours — enough time to infuse a part of this size.
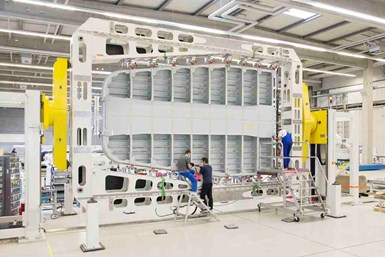
Step 7: A maneuverable jig holds the part in place for drilling and finishing steps, as the crossbeams, end wall and hinge are attached with metallic fasteners. Photo Credit: EADS Military Air Systems, Augsburg.
When the VAP process is initiated and a vacuum is pulled on the outer bag, the GORE-TEX allows air evacuation from each of the stringer bags. As resin is introduced, using vacuum pressure only, into each of the stringers as well as the skin, the GORE-TEX ensures that resin stays within each stringer bag for complete infusion. The company claims the special membrane makes the cocuring concept work. “The GORE-TEX allows air but not the resin to pass through, so we can ensure complete air evacuation and resin infusion of each stringer, maximizing final fiber content and minimizing voids,” says one engineer.
The outer skin/stringer part is then placed in an oven for several hours at 180°C/350°F to cure. No postcure is required.
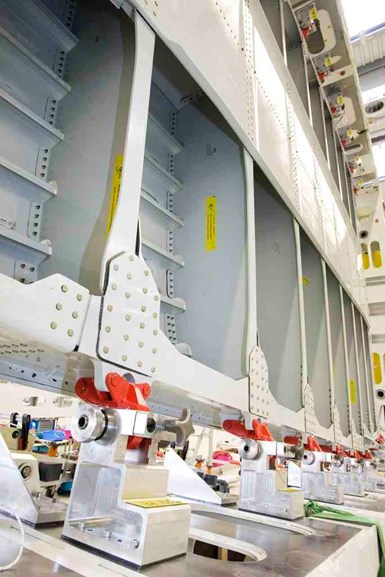
Step 8: A closeup of the painted part shows the metallic fasteners holding the crossbeams to the outer plate and to the end wall.Photo Credit: EADS Military Air Systems, Augsburg.
The door’s narrow inner skin is made in the same manner, using the same procedure to cocure its integral stringers. The remaining components are also made using VAP. The endwall incorporates sections of Rohacell PMI (polymethacrylimide) foam core supplied by Rohm Degussa GmbH (Darmstadt, Germany). The core is placed in the areas between crossbeam fastener locations, for less part weight.
The crossbeams are thick, solid laminates with a series of cutouts along their lower edge to accommodate the stringers. The beams also are made with spread flanges along their lower edges to facilitate through-bolting to the outer skin (see Step 8, at right).
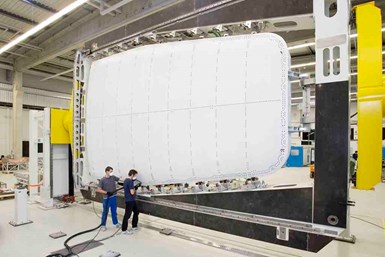
Step 9: The outer surface of the completed door. Photo Credit: EADS Military Air Systems, Augsburg.
Production ready
Assembly of the complete cargo door involves a large holding jig (see Step 9, at right), which supports the outer skin and enables assembly, bonding, drilling and fastening of all of the door’s components and attachment of the hinge parts. After assembly is complete, the doors are painted and delivered to Airbus in Bremen, Germany. There, as HPC went to press, three fuselages had been assembled, one of which will undergo static and dynamic testing to failure, according to Airbus.
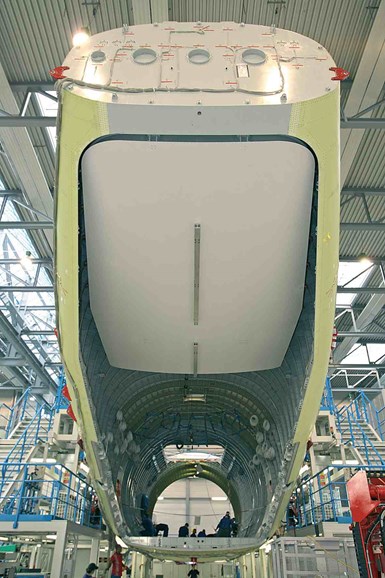
Step 10: The first door installed on an A400M rear fuselage. Photo Credit: Airbus Military.
Augsburg engineers note that, when in full production, at least three doors per month will have to be produced at the Augsburg facility. While hand-preforming methods sufficed for the initial articles, the company has contracted with a German company to produce a machine intended to automate the stringer preforming process, which will help make the infused part more cost-effective.
The first flight of the A400M is scheduled for January 2008. With more than 190 firm orders, Airbus is keen to meet its published schedule and begin deliveries of the plane to customers in the fall of 2009. A lot is riding on the success of the A400M — a new entry with the potential to rejuvenate the strategic airlift market.
Related Content
The state of recycled carbon fiber
As the need for carbon fiber rises, can recycling fill the gap?
Read MoreOne-piece, one-shot, 17-meter wing spar for high-rate aircraft manufacture
GKN Aerospace has spent the last five years developing materials strategies and resin transfer molding (RTM) for an aircraft trailing edge wing spar for the Airbus Wing of Tomorrow program.
Read MoreThe lessons behind OceanGate
Carbon fiber composites faced much criticism in the wake of the OceanGate submersible accident. CW’s publisher Jeff Sloan explains that it’s not that simple.
Read MoreCryo-compressed hydrogen, the best solution for storage and refueling stations?
Cryomotive’s CRYOGAS solution claims the highest storage density, lowest refueling cost and widest operating range without H2 losses while using one-fifth the carbon fiber required in compressed gas tanks.
Read MoreRead Next
Composite wing spars carry the enormous turboprop engines
Airbus A400M aircraft prompts new spar design and new manufacturing system designed to make it.
Read More“Structured air” TPS safeguards composite structures
Powered by an 85% air/15% pure polyimide aerogel, Blueshift’s novel material system protects structures during transient thermal events from -200°C to beyond 2400°C for rockets, battery boxes and more.
Read MoreCombining multifunctional thermoplastic composites, additive manufacturing for next-gen airframe structures
The DOMMINIO project combines AFP with 3D printed gyroid cores, embedded SHM sensors and smart materials for induction-driven disassembly of parts at end of life.
Read More