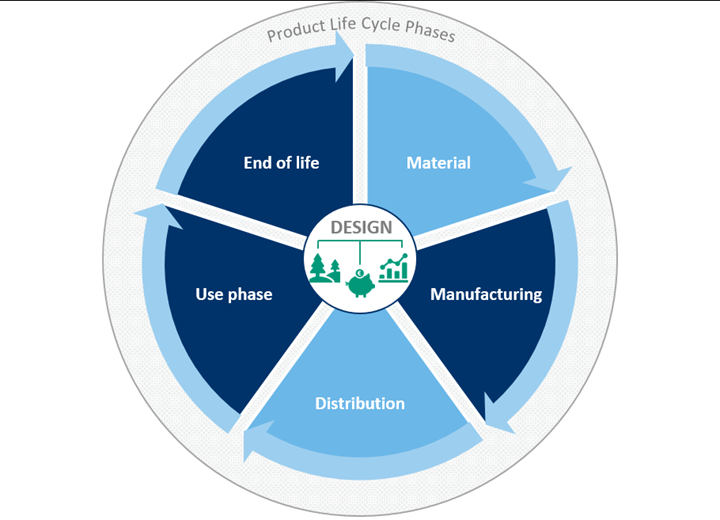
Figure 1: The design of a product results from the various requirements placed on the product itself along the product life cycle, from the choice of material to the end of its life. The design of a product therefore is the most important product development stage for a holistic optimization of the overall balance of a product regarding sustainability, economic and performance aspects. In the field of lightweight construction, manufacturing, the use phase and end-of-life (highlighted in dark blue above) have the greatest impact on the overall balance in the presented example. Photo Credit, all images: Fraunhofer IGCV
Biomimetic design has become an important approach in the quest for more sustainable composite products. However, the systematic transfer and application of materials, structures and principles from nature to products and industry requires a paradigm shift from constant efficiency optimization and maximum growth to sustainable strategies, long-term efficiency and robustness. In addition to new material and product properties, this also requires new production architectures and changes in product development, processes and planning. Model-driven design and analysis serves as a powerful tool to facilitate this transformation and enables controlled management of complex interdependencies along the life cycle of a product.
Achieving a balanced design
Lightweight construction aims to increase resource efficiency by reducing the mass of components. However, lightweight materials like fiber-reinforced polymers (FRPs) or high-performance metal alloys often have a relatively unfavorable ecological footprint in production and disposal. Aiming for a favorable overall balance, environmental accounting as part of design studies along the whole life cycle of a product is crucial, particularly in the manufacture, use and end-of-life (EOL) phases of a product (Fig. 1). However, because of complex dependencies between these phases, and the fact that each comes with a distinct set of parameters and optimization tasks for its evaluation, achieving a balanced design is not that simple.
Results of the manufacturing phase largely depend on an accurate prediction of material demand and the availability of valid manufacturing process models. For the use-phase model, contributions are determined by the mission scenario, service life and assumed overall system efficiency —consider, for example, an aircraft — as well as the allocation formula of the system’s energy consumption to its components. Contribution of the EOL phase hinges on the prospective disposal or recycling process and whether or not credits for recycled value streams are awarded.
To identify an optimal part design in terms of sustainability measures, all relevant design variants have to be evaluated. This becomes possible by the use of model-driven design synthesis of lightweight components in combination with life cycle assessment (LCA) and life cycle cost assessment (LCCA) tools. Specifically, the Fraunhofer Institute for Casting, Composite and Processing Technology (Fraunhofer IGCV, Augsburg, Germany) develops model-driven design workflows for lightweight design with the help of a graph-based design language implemented in the commercial software tool suite Design Cockpit 43, developed by the engineering firm IILS mbH (Leinfelden-Echterdingen Germany). The underlying design methodology is being developed at the University of Stuttgart (Stuttgart, Germany) and IILS.
Zeroing in on the best for each purpose
Considering an example in urban air mobility (UAM), a modular design program refines the component model step by step, and is driven by physical or heuristic design rules. A design task is specified through a set of functions and requirements, such as mechanical loads. A solution principle, such as a load-carrying beam, implements the specified functions. Thereafter, design steps like shape selection, material selection, sizing and detail design (production-specific) are executed automatically. Thanks to a modular architecture of the design sequence, many different design aspects can be implemented in each of these steps (Fig. 2). The design sequence is executed automatically, so that multiple variants can be generated without manual engineering efforts.
Figure 2: This graph depicts the systematic design sequence for lightweight composite beams. Based on a design task and concept design, various shapes and materials are applied. At the detail design stage, the component is adapted to a specific composite manufacturing method and a detailed component model is generated. This model is subsequently evaluated with respect to performance, costs and environmental footprint.
Each variant is analyzed with respect to three competing design goals of lightweight design:
- Minimization of structural mass to increase payload and energy storage capacity.
- Minimization of LCCs to ensure economic viability.
- Minimization of the product environmental footprint (PEF) to foster environmental sustainability.
While computing the structural mass from the CAD models’ volume and the presumed material density, LCC factors in material and manufacturing costs are calculated from different production models, as well as the cost of energy in the use phase. A more detailed model could also consider maintenance efforts and costs of disposal. The PEF is calculated with a simplified, forward-looking LCA using the software solutions GaBi (Sphera, Chicago, Ill., U.S.) and OpenLCA (GreenDelta, Berlin, Germany). Both modules — LCCA and LCA — rely on coordinated data with the same input parameter set containing design and process parameters.
Figure 3: Pareto analysis of six product variants, comparing component mass, LCCs and product environmental footprint. Variant 6, applying a carbon fiber-reinforced polyetheretherketone (PEEK) composite material, offers the lowest component mass. Variant 5, applying a carbon fiber-reinforced polyamide 6 (PA6) composite material, offers the lowest life cycle costs and product environmental footprint.
Combining automated design and analysis, the characteristics of all generated design variants are compared in a Pareto analysis that estimates the benefit delivered by each action, then downselects the most effective actions. This helps engineers to identify variants that offer a favorable tradeoff between different criteria. This analysis is used to identify the most promising variants. For an in-depth analysis of a particular variant (Fig. 3), all design data — such as forces and moments, laminate stacking, CAD models, process chains, use models, etc. — are readily available.
Mastering the challenge
While the general approach is relatively straightforward and generates different designs and evaluates them automatically, there are some challenges. First and foremost, each model involved requires a set of parameters characterizing materials, processes, machines, etc., participating in the model. The discussed models currently process more than 50 independent design inputs and parameters, and the LCA and LCCA modules pull even more parameters from specific data sources. For every design variant computed, all of these parameters must be retrieved. However, not all of them are available at a sufficient level of confidence. Therefore, analysis of uncertainties can help to better understand the accuracy, precision and sensitivity of the computed product designs and evaluation results.
In order to gradually increase the accuracy and variability of the design and evaluation process, more and more parameters are needed, so that efficient data acquisition is key. This is where the presented method may profit from other digitalization and Industry 4.0 initiatives such as smart factories and digital twins that deliver large amounts of data and can be used to model the production and use phase more precisely.
Yielding the fruit
The design methodology presented here provides a framework for studying complex interdependencies in lightweight design with respect to the entire product life cycle. In this way, it is possible to evaluate various generated product variants with respect to the ecological and economic impact of applying bionic structures, bio-based materials or bio-inspired algorithms to a specific design problem. Thus, the presented approach and design tools make a significant contribution to biological transformation in manufacturing, which aims (by applying processes and principles from nature into technological systems) to generate sustainable added value and a resource-efficient economy, as well as minimizing waste production.
The resulting “biological” or “resource-saving” variants can be compared to conventional variants, in terms of sustainability and performance measures, without substantial manual engineering efforts, in order to verify whether they have a positive impact and meet the company’s requirements. This approach is transferrable to all kind of products and manufacturing processes in different sectors including commercial aviation, UAM and ground transportation. Furthermore, it is expandable for further optimization tasks, such as acoustic noise reduction and heat transfer optimization.
With this approach, one can identify numerous beneficial applications for sustainable lightweight structures — both as single components and assembled systems. This approach also enables engineering organizations to make informed decisions with respect to the myriad relevant dimensions of creating sustainable value in the designs they develop.
About the Author
Maximilian Holland
Max (M.Sc.) is a research associate at Fraunhofer IGCV, where he develops model-driven design and analysis methods for fiber-reinforced polymers, with a particular focus on manufacturing and lifecycle aspects. He graduated from the University of Stuttgart with a Master’s degree in aerospace engineering in 2018. During his studies he focused on automated design of lightweight structures with graph-based design languages. Before joining Fraunhofer in late 2019, he gathered several years of industrial experience at Tier 1 aerospace supplier Premium AEROTEC as a student and professional in 2011-2016 and 2018-2019
About the Author
Marion Früchtl
Marion (Dr. rer. nat.) is head of the cross competence field “Biological Transformation” at the Fraunhofer Institute for Casting, Composite and Processing Technology (IGCV) in Augsburg, Germany. Prior, she was employed as scientific advisor to the president and responsible for the presidential unit of the Fraunhofer-Gesellschaft. She holds a Ph.D. in atmospheric physics and chemistry with the focus on isotope fractionation effects in ozone photolysis from Utrecht University. She also holds a Master of Science in physical geography.
Related Content
Optimizing a thermoplastic composite helicopter door hinge
9T Labs used Additive Fusion Technology to iterate CFRTP designs, fully exploit continuous fiber printing and outperform stainless steel and black metal designs in failure load and weight.
Read MoreThe basics of composite drawing interpretation
Knowing the fundamentals for reading drawings — including master ply tables, ply definition diagrams and more — lays a foundation for proper composite design evaluation.
Read MoreAutomated robotic NDT enhances capabilities for composites
Kineco Kaman Composites India uses a bespoke Fill Accubot ultrasonic testing system to boost inspection efficiency and productivity.
Read MoreCarbon fiber, bionic design achieve peak performance in race-ready production vehicle
Porsche worked with Action Composites to design and manufacture an innovative carbon fiber safety cage option to lightweight one of its series race vehicles, built in a one-shot compression molding process.
Read MoreRead Next
“Structured air” TPS safeguards composite structures
Powered by an 85% air/15% pure polyimide aerogel, Blueshift’s novel material system protects structures during transient thermal events from -200°C to beyond 2400°C for rockets, battery boxes and more.
Read MoreDeveloping bonded composite repair for ships, offshore units
Bureau Veritas and industry partners issue guidelines and pave the way for certification via StrengthBond Offshore project.
Read MoreAll-recycled, needle-punched nonwoven CFRP slashes carbon footprint of Formula 2 seat
Dallara and Tenowo collaborate to produce a race-ready Formula 2 seat using recycled carbon fiber, reducing CO2 emissions by 97.5% compared to virgin materials.
Read More