Materials & Processes: Fiber formats for composites
Fibers used to reinforce composites are supplied directly by fiber manufacturers and indirectly by converters in a number of different forms, which vary depending on the application. Here's a guide to what's available.
Fibers used to reinforce composites are supplied directly by fiber manufacturers and indirectly by converters in a number of different forms, which vary depending on the application.
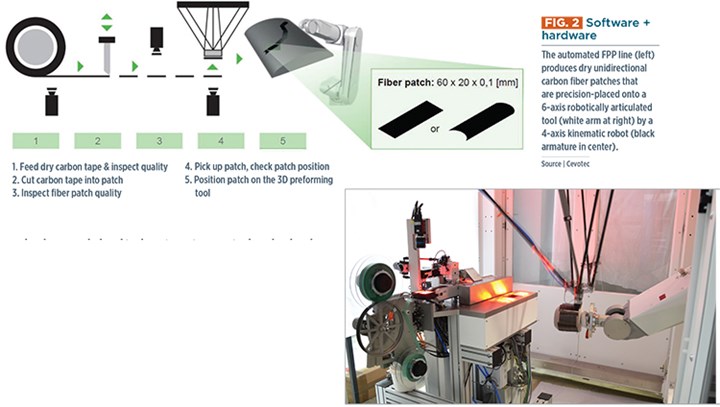
The automated FPP line (left) produces dry unidirectional carbon fiber patches that are precision-placed onto a 6-axis robotically articulated tool (white arm at right) by a 4-axis kinematic robot (black armature in center). Cevotec imports digital files prepared for specialty kiteboards into its own software to design local reinforcements that will meet both the kiteboard geometry and manufacturing process requirements as well as achieve the desired customer-specified performance improvements. Source: Cevotec
Roving and tow. Roving is the simplest and most common form of glass fiber. It can be chopped, woven or otherwise processed to create secondary fiber forms for composite manufacturing, such as mats, woven fabrics, braids, knitted fabrics and hybrid fabrics. Rovings are supplied by weight, with a specified filament diameter. The term yield is commonly used to indicate the number of yards in each pound of glass fiber rovings. Similarly, tow is the basic form of carbon fiber. Typical aerospace-grade tow size ranges from 1K to 24K (K = 1,000, so 12K indicates that the tow contains 12,000 carbon filaments). PAN- and pitch-based 12K carbon fibers are available with a moderate (33-35 Msi), intermediate (40-50 Msi), high (50-70 Msi) and ultrahigh (70-140 Msi) modulus. (Modulus is the mathematical value that describes the stiffness of a material by measuring its deflection or change in length under loading.) Newer heavy-tow carbon fibers, sometimes referred to as commercial-grade fibers, with 48K-320K filament counts, are available at a lower cost than aerospace-grade fibers. They typically have a 33-35 Msi modulus and 550-ksi tensile strength and are used when fast part build-up is required, most commonly in recreational, industrial, construction and automotive markets. Heavy-tow fibers exhibit properties that can approach those of aerospace-grade fibers but can be manufactured at a lower cost because of precursor and processing differences. (Carbon fiber's high cost and historically significant fluctuations in its supply and demand, generate perennially high interest in the composites industry about the state of the global carbon fiber market, a subject treated in the article "Supply and demand: Advanced fibers.")
A potentially significant recent variation is carbon fiber tow that features aligned discontinuous fibers. These tows are created in special processes that either apply tension to carbon tow at differential speeds, which causes random breakage of individual filaments, or otherwise cut or separate individual carbon filaments such that the filament beginnings and ends are staggered and their relative lengths are roughly uniform so that they remain aligned and the tow maintains its integrity. The breaks permit the filaments to shift position in relation to adjacent filaments with greater independence, making the tow more formable and giving it the ability to stretch under load, with greater strength properties than chopped, random fibers. Fiber forms made from aligned discontinuous tows (see “Mats,” below) are more drapable; that is, they are more pliable and, therefore, conform more easily to curved tool surfaces than fiber forms made from standard tow (see “Aligned discontinuous fibers come of age.”).
Mats are nonwoven fabrics made from fibers that are held together by a chemical binder. They come in two distinct forms: chopped and continuous strand. Chopped mats contain randomly distributed fibers cut to lengths that typically range from 38 mm to 63.5 mm. Continuous-strand mat is formed from swirls of continuous fiber strands. Because their fibers are randomly oriented, mats are isotropic — they possess equal strength in all directions. Chopped-strand mats provide low-cost reinforcement primarily in hand layup, continuous laminating and some closed molding applications. Inherently stronger continuous-strand mat is used primarily in compression molding, resin transfer molding and pultrusion applications and in the fabrication of preforms and stampable thermoplastics. Certain continuous-strand mats used for pultrusion and needled mats used for sheet molding eliminate the need for creel storage and chopping.
Woven fabrics are made on looms in a variety of weights, weaves and widths. Wovens are bidirectional, providing good strength in the directions of yarn or roving axial orientation (0º/90º), and they facilitate fast composite fabrication. However, the tensile strength of woven fabrics is compromised to some degree because fibers are crimped as they pass over and under one another during the weaving process. Under tensile loading, these fibers tend to straighten, causing stress within the matrix system.
Several different types of weaving are used for bidirectional fabrics. In a plain weave, each fill yarn (i.e., yarn oriented at right angles to the fabric length) alternately crosses over and under each warp yarn (the lengthwise yarn). Other weaves, such as harness, satin and basket weave, allow the yarn or roving to cross over and under multiple warp fibers (e.g., over two, under two). These weaves tend to be more drapable than plain weaves.
Woven roving is relatively thick and used for heavy reinforcement, especially in hand layup operations and tooling applications. Due to its relatively coarse weave, woven roving wets out quickly and is relatively inexpensive. Exceptionally fine woven fiberglass fabrics, however, can be produced for applications such as reinforced printed circuit boards.
Hybrid fabrics can be constructed with varying fiber types, strand compositions and fabric types. For example, high-strength strands of S-glass or small-diameter filaments may be used in the warp direction, while less-costly strands compose the fill. A hybrid also can be created by stitching woven fabric and nonwoven mat together.
Multiaxials are nonwoven fabrics made with unidirectional fiber layers stacked in different orientations and held together by through-the-thickness stitching, knitting or a chemical binder. The proportion of yarn in any direction can be selected at will. In multiaxial fabrics, the fiber crimp associated with woven fabrics is avoided because the fibers lie on top of each other, rather than crossing over and under. This makes better use of the fibers’ inherent strength and creates a fabric that is more pliable than a woven fabric of similar weight. Super-heavyweight nonwovens are available (up to 200 oz/yd²) and can significantly reduce the number of plies required for a layup, making fabrication more cost-effective, especially for large industrial structures. High interest in noncrimp multiaxials has spurred considerable growth in this reinforcement category.
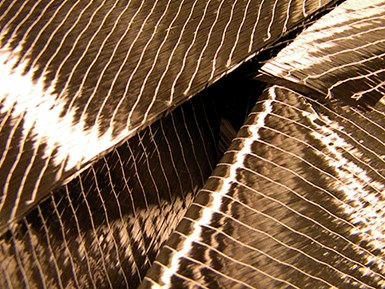
Relatively new, thin, biaxial reinforcements called C-PLY (pictured here) were used to form the quarter-scale VX-1 KittyHawk UAV's airframe, which features wings that smoothly blend into an airfoil-shaped fuselage. Layup for the VX-1 KittyHawk airframe's top and bottom skins used +45°/0° and -45°/0° C-PLY, alternating in a six-layer stack (12 plies per six layers), with a 0.006-inch thickness per two-ply layer. Source: VX Aerospace
A new style of multiaxial reinforcement, developed by Dr. Stephen Tsai of Stanford University together with Chomarat (Le Cheylard, France and Anderson, SC, US), was introduced in 2011 that orients fibers at very shallow angles, such as 0°/20°, that can replace quasi-isotropic fiber orientations for better performance and lower weight. One result is a product called C-PLY, recently used by VX Aerospace (Morganton, NC, US) on its quarter-scale VX-1 KittyHawk UAV. It features wings that smoothly blend into an airfoil-shaped fuselage and is first aircraft to fly Tsai's anisotropic laminates, and its full-scale version is intended to serve in unmanned civilian or military capacities (see the image and cpaotion at left). Read more about the KittyHawk UAV and how its builders made use of this novel fiber form in "VX Aerospace: Small company, big performance."
Braided fabrics are continuously woven on the bias and have at least one axial yarn that is not crimped in the weaving process. The braid’s strength comes from intertwining three or more yarns without twisting any two yarns around each other. This unique architecture offers, typically, greater strength-to-weight than wovens. It also has natural conformability, which makes braid especially suited for production of sleeves and preforms (see “Preforms,” below) because it readily accepts the shape of the part that it is reinforcing, thereby obviating the need for cutting, stitching or manipulation of fiber placement. Braids also are available in flat fabric form. These can be produced with a triaxial architecture, with fibers oriented at 0°, +60°, -60° within one layer. This quasi-isotropic architecture within a single layer of braided fabric can eliminate problems associated with the layering of multiple 0˚, +45˚, -45˚ and 90˚ fabrics. Furthermore, the propensity for delamination (separation of fiber layers) is reduced dramatically with quasi-isotropic braided fabric. Its 0°, +60°, -60° architecture gives the fabric the same mechanical properties in every direction, so the possibility for a mismatch in stiffness between layers is eliminated.
In both sleeve and flat fabric form, the fibers are continuous and mechanically interlocked. Because all the fibers in the structure are involved in a loading event, the load is evenly distributed throughout the structure. Therefore, braid can absorb a great deal of energy as it fails. Braid’s impact resistance, damage tolerance and fatigue performance have attracted composite manufacturers in a variety of applications, ranging from hockey sticks to jet engine fan cases.
Preforms are near-net shape reinforcement forms designed for use in the manufacture of particular parts by stacking and shaping layers of chopped, unidirectional, woven, stitched and/or braided fiber into a predetermined three-dimensional form. Complex part shapes can be approximated closely by careful selection and integration of any number of reinforcement layers in varying shapes and orientations. Because of their potential for great processing efficiency and speed, a number of preforming technologies have been developed, with the aid of special binders, heating and consolidation methods and the use of automated methods for spray up, orientation and compaction of chopped fibers.
A recent and unusually creative example of automating preform manufacture is Cevotec's (Garching, Germany) Fiber Patch Placement (FPP) technology, an automated way to place preform "patches" of carbon fiber composition into otherwise less-expensive glass-fiber reinforced kiteboards built by North Kiteboarding (Oberhaching, Germany), as a means to address highly individualistic preferences in terms of 'board performance on the part of kiteboarding enthusiasts without radically increasing kiteboard pricing (see illustration/photo and caption at left). Read more about it by clicking on "Fiber patch preforms help tailor kiteboard performance."
Prepregs are resin-impregnated fiber forms, manufactured by impregnating fibers with a controlled amount of resin (thermoset or thermoplastic), using solvent, hot-melt or powder-impregnation technologies. Prepregs can be stored in “B-stage,” that is, a partially cured state, until they are needed for fabrication. Prepreg tape or fabric is used in hand layup, automated tape laying, fiber placement and in some filament winding operations (see the corresponding headings in the “Fabrication methods” segment of CW’s Sourcebook). Unidirectional tape (all fibers parallel) is the most common prepreg form. Prepregs made with woven fibers and other flat goods offer reinforcement in two or more dimensions and are typically sold in full rolls, although small quantities are available from some suppliers. Those made by impregnating fiber preforms and braids provide three-dimensional reinforcement.
Prepregs deliver a consistent fiber/resin combination and ensure complete wetout. They also eliminate the need to weigh and mix resin and catalyst for wet layup. For most thermoset prepregs, drape and tack are “processed in” for easy handling, but they must be stored below room temperature and have out-time limitations; that is, they must be used within a certain time period after removal from storage to avoid premature cure reaction. Thermoplastic prepregs need no refrigeration and are not subject to outlife limitations, but without special formulation, they lack the tack or drape of thermoset prepregs and, therefore, are more difficult to form.
That prepregs produce finished parts with the lowest mass, highest mechanical properties and low void content is undisputable. However, they also have been, historically, the most expensive, in part, because they have, historically, been produced by specialists — the production of prepreg has been an intermediate, discrete step in the composites supply chain. Recently, efforts have been made to address the inefficiencies and associated costs related to this additional step. Two interesting approaches toward this end, both inline processes, were introduced at SPE’s Automotive Composites Conference & Exhibition 2015 in Detroit, MI, US. They turn composites fabricators into prepreggers in much the same way the direct long-fiber thermoplastic (D-LFT) process did in the late 1990s/early 2000s when the work of compounders was shifted to fabricators. Both new technologies eliminate the previously necessary and expensive steps of freezing and storing prepreg prior to shipping it to the customer, who then must also store it and thaw it before using it in a molding process, the costs of which are borne by the processor and, presumably, the processor’s customer.
Closest to commercialization is an inline prepregging process developed jointly by Mitsubishi Rayon Co. Ltd. (Tokyo, Japan) and Mitsubishi Rayon Carbon Fiber and Composites Inc. (Irvine, CA, US). Mitsubishi scientists reduce costs by directly coating single bundles of carbon tow, calibrating the width and then rewinding the product onto spools. An automated fiber placement (AFP) system — Mitsubishi calls it automated towpreg placement — is then used to lay up ply stacks, to eliminate the labor of hand layup. Stacks are subsequently preformed and molded via the company's own prepreg compression molding (PCM) process. A different approach is the new InPreg (inline-prepreg) process developed by the Fraunhofer Institute for Chemical Technology (ICT) (F-ICT, Pfinztal, Germany). Like Mitsubishi's PCM approach, InPreg prepregs are designed to be formed in compression presses rather than more exotic equipment, thus opening up laminate composites to a broader range of processors. the InPreg preforming and molding steps are both accomplished in the compression tool. This eliminates not only the time needed to heat, preform and cool prepreg, but also the cost and space for a preforming station. Key to the Inpreg process is a four-part, B-stageable epoxy resin system from Huntsman Advanced Materials (Basel, Switzerland) and lower cost 24-50K tow carbon fiber, which is formed into a UD, non-crimp fabric (NCF). (Read more about both inline methods in “Lower cost, less waste: Inline prepreg production.”)
Spread tow is an individual tow (or untwisted yarn) of fiber that has been spread out until the individual filaments lie side-by-side, forming an ultra-thin ribbon. For example, a 12K tow of carbon fiber may be spread from 5 mm to 25 mm in width, reducing its thickness by 80%. These spread tows can be woven into fabric, placed to form a multiaxial noncrimp fabric (NCF) or receive liquid or powder resin to form a spread-tow tape or towpreg. Use of woven spread-tow fabric in place of more conventional reinforcements can result in a 20-30% weight savings in the composite laminate. This is achieved by closing the warp and weft interstitial gaps between warp and weft so that less resin is trapped there, but also by reducing the fiber crimp, resulting in straighter fibers, which boosts strength. Thus, the final composite laminate may use fewer, thinner plies to achieve the same or better performance.
Fiber supplier Hexcel (Stamford, CN, US), claims 5-8% reductions in fabric gaps and the ability to achieve, with carbon fiber, 6K tow properties with 3K tow areal weight, 12K tow properties with 6K tow areal weight, etc. North Thin Ply Technology (NTPT, Penthalaz-Cossonay, Switzerland) claims that any fiber can be spread and claims that very low areal weights are achievable: 30 g/m2 for PAN-based carbon fiber and 14-micron diameter quartz fiber, 35 g/m2 for 9-micron diameter glass fiber, 20 g/m2 for aramid fiber and 30 g/m2 for polybenzoxazole (PBO) and other synthetic fibers. Suppliers of spread tow reinforcements include Hexcel, NTPT, Oxeon (Boras, Sweden), Sigmatex (UK) Ltd. (Runcorn, UK), Chomarat and FORMAX (Leicester, UK). Applications include bicycles, skis, hockey sticks, rackets, sailboats, racecars and the Solar Impulse aircraft.
Recycled carbon fiber (RCF) reinforcements are available in a variety of forms, including chopped fibers cut to specific lengths, chopped fibers compounded as long fiber thermoplastic (LFT) pellets, three-dimensional net-shaped preforms, and randomly oriented chopped fiber mats — either dry or combined with thermoplastics — including polypropylene (PP), polyethylene terephthalate (PET), polyamide (PA or nylon), polyphenylene sulfide (PPS), polyetherimide (PEI), polyetheretherketone (PEEK). The chopped fiber mats also can be processed — for example, via carding — to achieve greater fiber alignment, resulting in better mechanical properties. This variety of products is available from a range of RCF suppliers worldwide, and are recycled using pyrolysis, which burns resin from waste prepreg and cured structures. Technical Fibre Products Inc. (TFP, Schenectady, NY, US and Burneside, UK) makes veils from RCF as light as 2 g/m2.
RCF products are also made in-house from dry fiber manufacturing waste. SigmaRF products reuse Sigmatex’ in-house dry manufacturing waste by combining 45-mm to 60-mm carbon fibers with a thermoplastic carrier to form slivers which are used to make noncrimp fabrics, for example, a 220 g/m2 ±45° carbon fiber/PET biaxial NCF. Other variations include RCF/Kevlar/PEI, RCF/PA and RCF/PES.
The Institute of Plastics Processing (IKV) at RWTH Aachen University (Aachen, Germany) has taken nascent fibers not collected by rollers during carbon fiber PAN precursor spinning — a carbon fiber production waste or byproduct — and then chopped, carbonized and formed these into homogeneous mats using a continuous airlay process. (Read more about the technologies for reclaiming carbon fiber and the market for recycled product in “Recycled carbon fiber update: Closing the CFRP lifecycle loop.”)
New methods are also being developed to produce continuous recycled fibers including solvolysis using alcohols or other solvents to remove resins without burning or high temperatures, pyrolysis and unwinding of filament wound pressure vessels, and use of epoxy resins that enable the matrix to be recycled as a thermoplastic, such as Recyclamine hardeners by Connora Technologies (Hayward, CA, US).
Molding compounds are yet another way to incorporate fibers into a composite. Traditionally, these have been developed by the plastics industry and feature short fibers (2-25 mm) at low weight percentages (5-50%). Putty-like bulk molding compound (BMC) is used in injection molding while sheet molding compound (SMC) is used for larger parts and higher strength requirements, typically in a compression molding process.
Glass mat thermoplastic (GMT), also a compression moldable material, has continuous random-fiber reinforcement. GMT was developed in the 1960s as a step up from short fiber-reinforced nylon. It has faced increasing competition from long fiber-reinforced thermoplastic (LFRT or LFT) which is produced by cutting small-diameter pultruded continuous glass fiber rods into pellets. LFT features continuous unidirectional fiber running the full length of the pellet and offers properties between GMT and short-glass thermoplastics. In the 1990s, machinery OEMs developed inline compounding (ILC) systems that integrate the previously separate compounding and molding processes. These direct long-fiber thermoplastic (D-LFT) systems combine resin, reinforcement and additives at the press, delivering a measured shot or charge directly to injection or compression molding equipment. This eliminates inventories of pre-compounded product and enables tailored fiber length.
SMC, BMC, GMT and LFT are used in a wide range of applications where complex shapes and molded details are required, including automotive parts, appliances (washing machine tub), medical devices, consumer goods, electronics, sporting goods, brackets, enclosures, parts for transportation vehicles and electrical applications.
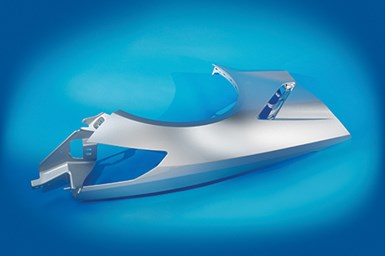
Low-density SMC: After five years of R&D at tier 1 automotive supplier Continental Structural Plastics (CSP, Auburn Hills, MI, US), judges at two recent industry events agreed that CSP's TCA Ultra Lite sheet SMC (1.2 specific gravity), used to mold, for example, this very complex, one-piece Corvette right-front fender, is a winner. The CAMX 2015 steering committee gave it the Unsurpassed Innovation award during its October conference in Dallas, TX, US, and a month later, it topped the SPE Automotive Division’s Materials category and was the Grand Award winner at the 45th SPE Automotive Innovation Awards Gala in the Detroit suburbs. Source: SPE Automotive Div.
SMC, in particular, offers parts-consolidation, deep-draw contour and a host of other benefits vs. steel and aluminum: It's typically 40% lighter than metals in specification-comparable geometries. Although it won't rust or corrode and doesn't need such treatment, it has the thermal and chemical resistance to survive automakers’ electrophoretic (e-coat) deposition rust prevention processes on metallic chassis components, so SMC parts can be attached to the body-in-white (the preferred assembly method) and don't require special post e-coat assembly. Until recently, however, SMC held a cost advantage at production volumes of 150,000 units or less. However, a new, low-density SMC from Continental Structural Plastics (CSP, Auburn Hills, MI, US) called TCA (tough Class A) Ultra Lite. At a specific gravity (SG) of 1.2, it offers a 28% mass reduction vs. CSP’s mid-density TCA Lite (1.6 SG) grades, and a 43% reduction vs. conventional 1.9 SG grades of SMC. Further, it not only offers mechanical performance comparable to TCA Lite (both feature a matrix of unsaturated polyester from AOC Resins, Collierville, TN, US), but also reportedly bonds more effectively to paint and adhesive. Most importantly, lifecycle analyses done by CSP reportedly show that even at volumes as high as 350,000-400,000 vehicles per year, TCA Ultra Lite costs less per part than aluminum (see the photo and caption at left.) Read more about the new SMC in "Low-density SMC: Better living through chemistry."
Glass fiber is the most common and least expensive reinforcement used in molding compounds, aramid fiber provides wear resistance, stainless steel fiber achieves both electrostatic dissipation (ESD) and electromagnetic interference (EMI) shielding, while carbon fiber provides higher modulus and lower weight as well as ESD properties. Molding compounds reinforced with natural fibers (hemp, flax, sisal and wood-derived fibers) also have been developed, including. These are gaining popularity in automotive, sporting goods and consumer products.
Advanced molding compounds are aimed at higher performance applications including aerospace and military parts. These materials use higher performance resins, such as epoxy, phenolic, vinyl ester, bismaleimide (BMI) and polyimide, and fiber loadings from 45% to 63% by weight. Fibers include carbon and E-glass, but also higher performing S2-glass. TenCate Advanced Composites BV (Nijverdal, The Netherlands) makes BMCs with epoxy, cyanate ester, Nylon, PPS or PEEK resins and carbon or S2-glass fiber in lengths from 12 mm to 50 mm. HexMC is produced by Hexcel, using 50 mm long carbon fibers and epoxy resin. A variety of other carbon fiber SMC products are available from suppliers that include Continental Structural Plastics, Quantum Composites Inc. (Bay City, MI, US) and a joint venture between Zoltek Corporation (St. Louis, MO, US) and Magna Exteriors (Paris, France).
Recently, molding compounds have been enabling reinforcement of products created by means of what have come to be known as additive manufacturing processes, also known as 3D printing. Chopped and short-fiber reinforcement can be adapted for use in oen type of 3D Printing, called Fused Deposition Modeling. Most 3D printing of reinforced plastics is limited in size (for an overview, see “3D Printing: Niche or next step to manufacturing on demand?”). But at least one recent demonstration project, indicates that large-format printing is technically practical and economically justifiable: Oak Ridge National Laboratory (Oak Ridge, TN, US) and machinery builder Cincinnati Inc. (Harrison, OH, US) demonstrated large-format printing capability with the Big Area Additive Manufacturing (BAAM) system, working with Local Motors (Chandler, AZ, US) to produce the world’s first 3D-printed automobile body. The custom-designed Strati sports car body was printed on the exhibit floor at the 2014 IMTS show in 44 hours, using a 15% carbon fiber reinforced acrylonitrile butadiene styrene (ABS) compound supplied by SABIC (Pittsfield, MA, US). Read more about the demonstration in “Additive manufacturing: Can you print a car?”
Editor's note: To continue reading articles in "Industry Overivew, Part I: Materials & Processes," you may return to the SourceBook main menu by clicking here.
Related Content
Infinite Composites: Type V tanks for space, hydrogen, automotive and more
After a decade of proving its linerless, weight-saving composite tanks with NASA and more than 30 aerospace companies, this CryoSphere pioneer is scaling for growth in commercial space and sustainable transportation on Earth.
Read MoreRTM, dry braided fabric enable faster, cost-effective manufacture for hydrokinetic turbine components
Switching from prepreg to RTM led to significant time and cost savings for the manufacture of fiberglass struts and complex carbon fiber composite foils that power ORPC’s RivGen systems.
Read MoreJEC World 2023 highlights: Recyclable resins, renewable energy solutions, award-winning automotive
CW technical editor Hannah Mason recaps some of the technology on display at JEC World, including natural, bio-based or recyclable materials solutions, innovative automotive and renewable energy components and more.
Read MoreDrag-based wind turbine design for higher energy capture
Claiming significantly higher power generation capacity than traditional blades, Xenecore aims to scale up its current monocoque, fan-shaped wind blades, made via compression molded carbon fiber/epoxy with I-beam ribs and microsphere structural foam.
Read MoreRead Next
Lower cost, less waste: Inline prepreg production
New technologies move direct (fabricator-based) prepregging closer to reality.
Read MoreAligned discontinuous fibers come of age
Discontinuous but aligned carbon fibers are proving formable and formidable in high-performance, compound-curvature applications.
Read More3D Printing: Niche or next step to manufacturing on demand?
With and without fiber reinforcement, additive manufacturing is making an impact, but to what end?
Read More