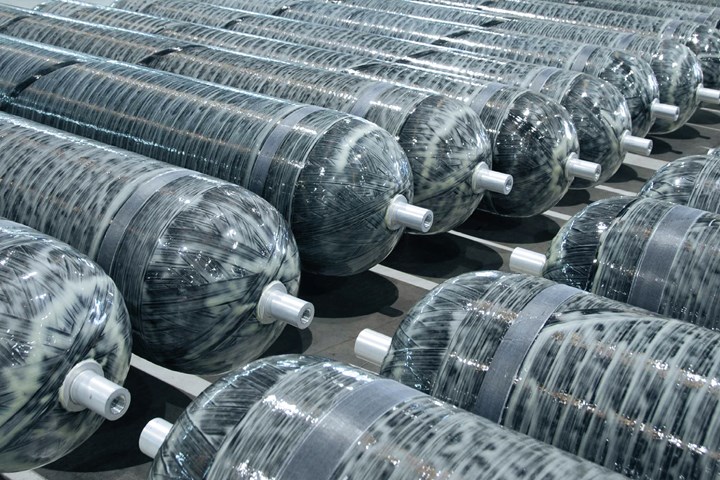
Cummins joint venture with NPROXX
Investment in H2 is increasing. Type IV tank manufacturer NPROXX is now a 50/50 joint venture with Cummins, which has also acquired key suppliers of fuel cells and H2-producing electrolyzers. These 500-millimeter-diameter, 2,200-millimeter-long automotive/heavy-duty tanks by NPROXX store H2 at 350 bar. Photo Credit: NPROXX
The significant projected growth in hydrogen vehicles that require Type IV, carbon fiber-reinforced polymer (CFRP) tanks to store hydrogen (H2) as a compressed gas was explored in part 1 of this series. H2 can be combusted directly or used in a fuel cell to produce electricity, with water and heat as the only emissions. As the world speeds up its transformation to zero-emission transport and industries, H2 is a key enabler. However, the very large amounts of carbon fiber needed to produce the millions of storage tanks required for these efforts may not be available in time to meet fuel cell vehicle (FCV) and infrastructure targets.
Other possible hurdles for Type IV tank growth include carbon fiber and CFRP tank cost as well as lower storage density versus cryotanks, which are mostly metal. New tank manufacturers and French automotive Tier 1 suppliers Plastic Omnium (Lavallois) and Faurecia (Nanterre) have set goals to cut Type IV tank cost by 30-75% by 2030 while boosting storage efficiency beyond 7%. As presented in CW’s Tech Days Hydrogen event in May, new technologies are being developed to aid these efforts, from Cevotec’s (Unterhaching, Germany) fiber patch placement (FPP) technology for cutting CFRP time and cost in tank domes, to Cygnet Texkimp’s (Northwich, U.K.) 3D winding for reduced fiber damage, to composite sensor integration specialist Com&Sens (Eke, Belgium) proposing in-situ tank monitoring.
Here in part 2, the additional markets of H2 refueling stations (HRS) and tube trailers for distribution are discussed, including the use of glass fiber for Type IV tanks. The issues of cost and storage efficiency, recertification of tanks and the use of sensors for this recertification as well as optimized production and health monitoring are also explored.
Hydrogen refueling stations
The ambitious road maps for H2-powered cars and trucks will require building a large number of HRS. A Feb 2021 report by H2stations.org noted 107 HRS went into operation in 2020, the highest one-year total to date. With seven more added in Jan 2021, 560 HRS now operate globally, with plans already in place for an additional 225 stations. The Hydrogen Council’s Feb 2021 “Hydrogen Insights” report estimates that 10,300 HRS will be needed by 2030 to meet FCV targets.
CFRP tanks are being used in HRS. For example, Hexagon Composites (Alesund, Norway) supplied tanks to Resato (Assen, Netherlands) for a large HRS in The Hague, and NPROXX sold tanks for two HRS to regional bus operator RVK (Cologne, Germany).
Fig. 1. Elements of a hydrogen refueling station (HRS)
Schematic of elements comprising a hydrogen refueling station (HRS). Type IV composite pressure vessels may be used in tube trailers to deliver H2 to the station and/or in the cascade of tanks for buffer storage on site. Photo Credit: CW
Hydrogen is supplied to an HRS by tube trailer, liquid tanker or pipeline or it can be generated on site using a steam methane reformer (SMR) or electrolysis unit (Fig. 1). Type IV CFRP tanks may be used in the tube trailers to deliver H2 and also in the buffer storage on site.
In the May 2021 CW Tech Days event, PowerTap Hydrogen (Irvine, Calif., U.S.) COO Kelley Owen explained that each PowerTap HRS will produce 1,250 kilograms of H2 per day from natural gas using an SMR unit to refill FCV equipped with 350-bar and 700-bar tanks. Partnered with Andretti Group, PowerTap will install HRS at selected Andretti Group gas and diesel stations in California.
Fig. 2. Onsite storage space at a premium
Rough design of an HRS using a steam methane reformer and Type 1 steel tanks for buffer storage, requiring 25 x 35 feet of storage space. Photo Credit: PowerTap Hydrogen
These HRS units must fit within the current station footprint, already occupied by gas and diesel pumps, convenience stores, etc. Owen said PowerTap’s buffer storage will comprise two sets of tanks: 24 medium-pressure (500 bar, 20-inch diameter, 34 feet long) and 30 high-pressure (900 bar, 16-inch diameter, 35 feet long) rolled steel pipe cylinders requiring approximately 25 x 35 feet of storage space (Fig. 2). Type I steel pipes were chosen, Owen explains, because Type IV composite tanks cost 30-35% more, increasing tank cost from $1.2 million to $1.6 million, which would consume more than half the typical $3 million budget per HRS.
“There’s no question that the price of steel tanks is much lower than that of composite tanks,” says Rick Rashilla, senior vice president of research and development for Hexagon Composites in Lincoln, Neb. “But there are some applications where composite tanks are a good solution. For example, some of our customers in Europe have mounted such tanks on top of the hydrogen station roof, compressors or other equipment to reduce the overall footprint. This is possible because the Type IV tanks are so lightweight. The metal tanks, though less costly, typically require a ground mount, and you can only stack them so high, due to their weight, whereas the composite tanks can be stacked higher, which reduces storage space.”
But are composite cylinders limited in diameter or pressure versus steel tanks? “The wall thickness of any pressure vessel has to increase as the pressure and size increase,” explains Brian Yeggy, principal engineer for Hexagon Composites R&D in Lincoln, Neb. “But there’s no limit within the composite technology that prevents us from making larger diameter tanks at 700 bar.” Currently, Hexagon’s largest tank is 580 millimeters/22.8 inches in diameter and 3.3 meters/10.8 feet long for stationary storage at 500 bar. It also has a 1,000-bar, 20-inch-diameter stationary storage cylinder. “That’s not a limitation of our technology, but just what has been demanded by our customers,” says Yeggy. “We’ve scaled composite tanks to 42 inches in diameter and 51 feet long for storage at 250 bar.”
“The higher the pressure,” he continues, “the more attractive composites look, but the more costly the system in total becomes, regardless of material, due to the higher wall thickness required.” And what about the ability for composite tanks to meet the needs of HRS for a 15-year life at up to 10 pressurization cycles per day? “That would equate to a maximum of 55,000 cycles,” says Yeggy. “Most of our products are already certified for 15 to 30 years per a standard of 45,000 cycles with proof fills to 150% overfill without failing. We’ve also tested several 27-inch-diameter, 379-bar tanks to 110,000 cycles without failure [Fig. 3], and we have a 14-inch diameter, 380-bar cylinder that has been tested to 1 million cycles, with each cycle at 150% of its working pressure. So, I have no concerns that Type IV tanks are capable of meeting the cycle range needed for hydrogen refueling stations.”
Fig. 3. Type IV tanks handle refueling cycles
Selected fatigue test data for Hexagon Composites Type IV compressed gas cylinders show composites’ ability to handle the fatigue of pressurization cycles, regardless of tank size and working pressure. Photo Credit: Hexagon Composites
H2 distribution and glass fiber
Tube trailers represent yet another market for composite cylinders—i.e., for the distribution or transport of hydrogen gas from point of production to an HRS or industrial site. This is a key market for Hanwha Cimarron Composites (Seoul, South Korea), Hexagon and NPROXX (Heerlen, Netherlands). At Hexagon, this market is expected to comprise >40% of the company’s 2021 revenue. “For hydrogen, most of our distribution cylinders are 350 bar and trending toward 500 and upward,” says Rashilla at Hexagon. “There will most likely be 700-bar cylinders as well, due to the need to get more hydrogen onboard; but it will always be a balance of how much it costs to get it there.”
“Everything starts in hydrogen with infrastructure,” says Michael Himmen, managing director and head of sales for NPROXX, explaining that growth in H2-powered vehicles requires refueling stations that in turn rely on fuel transport, “and we will probably present new solutions in terms of vessel and container designs in the next two years.” Here, the term “container” refers to how the transport modules are packaged. For example, Hexagon’s X-STORE modules for H2 transport at 500 bar comprise a 10-, 20-, 30- or 40-foot-long metal-framed trailer with 22, 52, 82 or 103 Type IV cylinders carrying a total of 240, 565, 890 or 1,115 kilograms of H2. NPROXX has similar offerings. Cimarron Composites explained in CW’s 2020 feature article on H2 pressure vessels that its Neptune 517-bar Type IV tanks, with a 30-inch diameter and 19-foot length, enable packing nine vessels into a standard 20-foot container for 600 kilograms of H2 and twice that amount in a 40-foot container.
Fig. 4. Type IV glass fiber tanks
Glass fiber composite Type IV tanks offer lower cost vs. carbon fiber composites, but less weight vs. steel (orange square in graph above). Photo Credit: UMOE Advanced Composites
Umoe Advanced Composites (UAC, Kristiansand, Norway) is also targeting H2 gas transport, but without carbon fiber. Instead, the company produces Type IV cylinders made using the industry-standard T4 plastic liner overwrapped with a filament-wound glass fiber/epoxy combination. UAC is currently supplying from 200- to 350-bar modules and will expand to include 450- and 500-bar tanks in 2022. As explained by Øyvind Hamre, CEO of UAC, in CW’s Hydrogen Tech Days event, the glass fiber-reinforced polymer (GFRP) tanks offer the same capital expenditure (CAPEX) as steel tanks but weigh 70% less (Fig. 4). Meanwhile, GFRP cuts CAPEX by 50% versus CFRP cylinders, though at a higher weight. In May 2021, UAC announced a joint venture to build a new factory in China that will scale production capacity from 10,000 to 20,000 cylinders (1,700-2,000 liters in size) per year by 2022. At the same time, it will increase its Norwegian capacity to 4,000 cylinders/year.
Cost and storage efficiency
As explained in the introduction of part 1 in this series, alongside cost, the other key issue for Type IV tanks is storage density — that is, how much H2 you can store in a given volume. The key metric here is termed gravimetric, weight or mass ratio, and is defined as the mass of H2 stored divided by the mass of the tank or storage system. A 2019 review of hydrogen storage systems listed gravimetric density for Type I, II and III tanks as 1.7%, 2.1% and 4.2%, respectively. This metric may also be referred to as storage density or efficiency. For Type IV tanks, the benchmark value of 5.7% was established by Toyota (Toyota City, Japan) in 2014 for its 700-bar tanks thanks to a 20% reduction in the CFRP used by optimizing fiber winding patterns. These typically comprise hoop winding along the cylinder, low-angle winding across the cylinder and domed ends and high-angle winding at the boundary between the hoop and low-angle windings. Toyota devised a method to eliminate high-angle helical winding, which previously comprised ≈25% of the laminate. Instead, the shape of the liner was flattened to enable lamination by hoop winding at the boundary regions. Toyota also concentrated hoop winding at the inner layers, where stress is highest. One of the advantages that Faurecia has claimed in its CFRP Type IV tanks is >7% weight ratio.
In 2019, NPROXX, now a 50/50 joint venture with Cummins (Columbus, Ind., U.S.), unveiled a 700-bar Type IV tank with a storage density of 6.4%, while the tanks Iljin Hysolus (Bongdong-eup, South Korea) produces for the Hyundai Nexo fuel cell passenger car average 6.3%. “For us, it's all about how you treat the carbon fiber, how much damage you do to your fibers during winding,” notes Himmen at NPROXX. “And that actually determines how much carbon fiber you use in the tank.”
H2 tanks made by Hexagon Composites, now spun off as Hexagon Purus, range between 5% and 7% storage efficiency, says Yeggy. “We're continually doing research to drive storage efficiency higher, but the more lightweight you make the tank, the more precise it has to be and the more damage tolerance you lose,” he notes. “And cost is a big factor. If cost is no concern, then I can build designs on paper that will push 10% storage efficiency. But to achieve that, it will cost more than the vehicle it’s in.”
Although reduced tank cost has been a goal pursued by numerous U.S. Dept. of Energy (DOE) programs for more than two decades, Faurecia claims it will reduce, by 2030, H2 storage system cost by 75%, from €1,300 to €315 per kilogram H2 stored. Plastic Omnium is targeting a more modest 30% cost reduction. NPROXX agrees with the latter. “Based on the larger volumes of tanks produced by 2030, our analysis is that we can drop cost by 25-30%,” says Himmen. However, he acknowledges that carbon fiber makes up 60% of the tank cost — and DOE cost models show that higher volumes do not significantly reduce fiber price. Hexagon has worked on various tank and carbon fiber cost reduction projects with the DOE, says Hexagon Composites’ Rashilla, and notes potential for additonal programs in the near future.
Recertifying and monitoring tanks
Rashilla points out another way to reduce costs is via Hexagon’s Digital Wave business unit, which uses modal acoustic emission (AE) to inspect tanks at the end of their regulatory life, analyzing tank integrity for additional service. Modal AE uses advanced electronics and sensors designed for increased sensitivity and data. “To extend the life of the cylinder is a great way to reduce costs and emissions,” says Rashilla. “This is already being demonstrated with carbon fiber composite cylinders for firefighters. We’ve also done this for our first production batches of compressed natural gas tanks that reached the end of their service life in 2010-2015. The Digital Wave testing can validate that a tank isn't damaged and it has ‘X’ amount of life remaining with high probability.”
“I think the industry is still lacking data,” says Himmen. “Type IV vessels are not inspected until after the first 10 years of service. But what has actually happened to the pressure vessel integrity in that time, and do you really have data to understand it? We are involved in an EU program to create this data. We have supplied a large number of tanks and equipped them with all kinds of sensors to determine the lifetime impact on tank integrity in terms of temperature, pressure cycles, aggressive fueling and in-service use and abuse. The goal is to gain more knowledge before we put sensors into the composite, because I first want to know what to measure and what to compare with to decide if the pressure vessel is good or bad.”
Future efforts notwithstanding, one area where NPROXX is currently integrating sensor technology is in its production process.
Fig. 5. Fiber optic sensors for optimized tank production, monitoring and design
Com&Sens uses fiber Bragg grating (FBG) fiber optic sensors embedded within or on the surface of composite tanks to sense strain and temperature during filament winding, testing and operation. These sensors can validate stress for FE models, provide structural health monitoring and tank integrity measurements for recertification. Photo Credit: Com&Sens
Com&Sens presented how embedded fiber Bragg grating (FBG) fiber optic sensors can be used to optimize composite tank production and in-situ health monitoring during CW’s H2 Tech Days. The FBG fiber optic line can be co-wound from a spool as the H2 tank laminate is being filament wound. Each fiber optic can range from 1-100 meters long and have up to 20 sensing points with a minimum of 1 centimeter spacing.
“Each sensing point is positioned within the optical fiber to measure at any discrete location within hoop or helical layers of the composite laminate,” says Com&Sens co-founder Eli Voet. “These points are interrogated during winding using a rotating joint, or during operations using a patented point connection technology that enables mass usage of ‘smart’ tanks.” He explains that the FBG embedded in the laminate senses the redistribution of strain inside the material. “These sensors can be used for production process optimization, allowing the understanding and digitizing of process parameters that could not be measured before,” Voet adds. “Capturing system-wide stress, strain and temperature measurements during curing, fatigue cycling and bursts tests will help designers to validate FE models and optimize, for example, material usage in future designs. But perhaps the most promising application is for lifecycle and structural integrity monitoring during tank operations, increasing acceptance and confidence in hydrogen technologies.” As an example, Voet presented the ability to produce tanks with a “digital thumbprint,” and then recertify the tank against that thumbprint for potentially extended service life.
Real change coming fast
According to the Hydrogen Council’s 2021 “Hydrogen Insights” report, 75 countries have zero-emission strategies and more than 20 countries have announced sales bans on internal combustion engine (ICE) vehicles before before 2035. In addition to H2, battery-electric, solar and other alternative fuels will be part of the solutions needed to meet these goals. However, there is a growing focus on and increased government support of H2. Net zero by 2050 has been the mantra for avoiding climate catastrophe, but many countries are pushing to hit targets by 2040 or sooner. Will this be possible?
“You have to rethink not only the vehicle architecture, but the whole business model of the operations,” says Dahl at Hexagon Purus Maritime. “Of course, there’s a kind of hesitance — who will be the first mover and take the risk? And there is also a lot of investment required. But those who have the goal, and the boldness to do it, will be the leaders going forward.”
In addition to multiple zero-emission solutions, there will also continue to be multiple solutions for H2 storage, and not all will rely on composites or carbon fiber. How will the composites industry respond to the rapidly emerging market for H2-powered vehicles, trains, ships and refueling stations being developed? Could new types of carbon fiber enable tanks with higher storage efficiency and lower cost? “We think a lot about the ideal carbon fiber we want to work with,” says Himmen at NPROXX. “Such larger volumes are projected, and obviously much higher consumption of carbon fiber [is also projected], and that ideal carbon fiber that I think about is not yet on the market.” But perhaps it could be. “You need to disrupt to make real change,” notes Dahl, “and 2050 is coming very fast.”
Related Content
Mikrosam, H2Storage collaborate on 300+-liter Type IV hydrogen tanks
Automated filament winding cell achieving wind speeds of 6 meters/second improves production performance, shortens curing cycle for serial production of 700-bar Type IV tanks.
Read MoreCollins Aerospace to lead COCOLIH2T project
Project for thermoplastic composite liquid hydrogen tanks aims for two demonstrators and TRL 4 by 2025.
Read MoreComposites end markets: Batteries and fuel cells (2024)
As the number of battery and fuel cell electric vehicles (EVs) grows, so do the opportunities for composites in battery enclosures and components for fuel cells.
Read MoreHexagon Purus opens new U.S. facility to manufacture composite hydrogen tanks
CW attends the opening of Westminster, Maryland, site and shares the company’s history, vision and leading role in H2 storage systems.
Read MoreRead Next
Hydrogen is poised to fuel composites growth, Part 1
Applications abound for composite tanks to store compressed H2 gas, but challenges exist in cost, size, efficiency and limited carbon fiber capacity.
Read MoreCFRP planing head: 50% less mass, 1.5 times faster rotation
Novel, modular design minimizes weight for high-precision cutting tools with faster production speeds.
Read MoreVIDEO: High-rate composites production for aerospace
Westlake Epoxy’s process on display at CAMX 2024 reduces cycle time from hours to just 15 minutes.
Read More